Microfiltration: Applications and potentialities in the dairy industry
- Like
- Digg
- Del
- Tumblr
- VKontakte
- Buffer
- Love This
- Odnoklassniki
- Meneame
- Blogger
- Amazon
- Yahoo Mail
- Gmail
- AOL
- Newsvine
- HackerNews
- Evernote
- MySpace
- Mail.ru
- Viadeo
- Line
- Comments
- Yummly
- SMS
- Viber
- Telegram
- Subscribe
- Skype
- Facebook Messenger
- Kakao
- LiveJournal
- Yammer
- Edgar
- Fintel
- Mix
- Instapaper
- Copy Link
Posted: 27 February 2013 | Geneviève Gésan-Guiziou, Research Director at the French Institute for Agricultural Research, INRA | No comments yet
Membrane applications in the dairy industry date back to the late 1960s with the concentration of milk proteins using the MMV process named after its inventors, Maubois, Mocquot and Vassal. Since then, membrane applications in the dairy sector have not stopped growing and nowadays, the presence of membrane equipments in dairy factories is very common. The equipment encompasses a large variety of different processes that have been successfully implemented throughout the diff – erent manufacturing stages, from reception of raw milk to waste-water treatment.
Membrane applications in the dairy industry date back to the late 1960s with the concentration of milk proteins using the MMV process named after its inventors, Maubois, Mocquot and Vassal. Since then, membrane applications in the dairy sector have not stopped growing and nowadays, the presence of membrane equipments in dairy factories is very common. The equipment encompasses a large variety of different processes that have been successfully implemented throughout the diff - erent manufacturing stages, from reception of raw milk to waste-water treatment.
Membrane applications in the dairy industry date back to the late 1960s with the concentration of milk proteins using the MMV process named after its inventors, Maubois, Mocquot and Vassal. Since then, membrane applications in the dairy sector have not stopped growing and nowadays, the presence of membrane equipments in dairy factories is very common. The equipment encompasses a large variety of different processes that have been successfully implemented throughout the diff – erent manufacturing stages, from reception of raw milk to waste-water treatment.
Among the different membrane technologies encountered in this sector, microfiltration (MF) is a very attractive separation process. It offers various fractionation possibilities of milk components and makes it possible to ensure the safety of dairy products and to create products with high added value and new functionalities.
General aspects of microfiltration
The membranes used in MF have a porous structure with pore sizes ranging from approximately 0.1μm to a few micrometres. The representation of the milk components versus their diameter expressed in a logarithm scale shows that it is possible to use these membranes for the retention / separation of various kinds of milk components such as casein micelles, fat globules, somatic cells and particles / aggregates either naturally present or formed by previous pretreatments (Figure 1, page 61).
For instance, MF makes it possible to separate bacteria for skimmed milk provided the fat has previously been removed because the size distribution of fat globules is similar to that of bacteria. This technology is also particularly adapted to the separation of casein micelles from soluble proteins provided that milk is not heat treated because heat denaturation of soluble proteins leads to aggregation and change in size.
In the dairy industry today, membranes are often ceramic membranes in tubular configura – tion. With this kind of membrane, crossflow velocity or wall shear stress is classically high and transmembrane pressure (ΔP) is low. The high crossflow velocity is required in order to erode accumulated particles at the membrane surface, but results in high pressure drop in the retentate compartment. Simultaneously, ΔP must be low in order to operate in what we call the pressuredependent region that should be favoured for optimal production (Figure 2). Indeed, at a given crossflow velocity, J increases linearly with increasing pressure, usually up to a limiting value above which the permeate rate becomes pressure-independent and does not increase anymore, due to the accumulated layer or the gel formed at the membrane surface. In that pressure-independent region, an increase of pressure (that is directly related to the energy consumption) does not lead to a significant increase of flux. This region should then be avoided due to high fouling, difficulties of cleaning and high energy consumption.
The difficulty in MF systems consists then in operating separation in the pressure-dependent region with a high retentate pressure drop (induced by crossflow velocity). The high retentate pressure drop leads to high fouling heterogeneity and is incompatible with low and homogeneous transmembrane pressure along the filtering path. To overcome this phenomenon and make it possible to perform MF with simultaneous high crossflow velocity and low transmembrane pressure, specific equipments have been developed. Today, most MF plants in dairies either operate according to the hydraulic concept of the uniform trans – membrane pressure (UTP) patented by Alfa- Laval under the trademark Bactocatch1 or use membrane with linear gradient of resistance.
In the UTP system, the MF permeate is circulated in co-current to the MF retentate in order to create a permeate pressure drop similar to retentate pressure drop. The permeate pressure drop leads to a constant trans – membrane pressure that leads itself to a constant flux through the membrane. Obviously this system requires an extra pump in the permeate side.
The other alternative is the use of a specific membrane. The objective consists then in creating an inhomogeneous membrane, having a higher hydraulic resistance at the membrane entrance where the local transmembrane pressure is high, and a low resistance at the membrane outlet. This membrane concept makes it possible to obtain homogeneous filtration performance all along the membrane length without permeate circulation loop, avoiding consequently extra investment and running costs due to the permeate pump. Two types of membranes are commercially available: the Membralox® GP membrane from Exekia-Pall2 and the Isoflux® membrane from Tami Industries3. Both are obviously constructed for well-defined pressures and hydrodynamic conditions, and consequently must be used for well-defined applications.
Such equipment has been largely used in the dairy industry and lead to satisfactory performance but, because of the high running costs of ceramic membrane plants, the industry has recently started to operate MF with an organic membrane in a spiral wound con – figuration. Spiral-wound membranes are actually considered very attractive because of their relative low running and investment cost, despite their low performance mainly attributed to fouling heterogeneity and their limitations in terms of acceptable pH range, chlorine resist – ance and temperature range.
Main applications of MF in the dairy industry
In terms of applications, MF has been developed at industrial scale for two main applications: removal of bacteria from milk and selective separation of casein micelles from soluble proteins. The MF area can be estimated to reach 15,000m2. This is far-removed from ultra – filtration, which is widely used mainly for milk and whey protein concentration.
Removal of bacteria
Like most food fluids, milk and its derivates provide a favourable media for spoilage microorganisms, which can lead to alteration of chemical composition of milk and difficulties in milk preservation and transformation. In that context, MF consists in removing bacteria from milk in order to minimise possible health hazards and control bacteria growth during milk processing. It offers then an interesting alternative to heat-treatment or centrifugation4. At industrial level, milk is generally skimmed before MF because the size of bacteria overlaps the size of fat globules (Figure 1 and Figure 3, page 62). The skimmed milk is then microfiltered, and the cream, classically treated at about 120°C for four seconds to eliminate bacteria, is added back to the microfiltered skimmed milk. The micro filtration retentate, which contains most of the bacteria, can be discharged separately for other suitable applications or blended continuously with the cream. In order to reduce the volume of the retentate, a second MF stage can also be added. MF is classically performed with multichannel ceramic membrane with a pore size of around 0.14 μm, at temperatures between 35 and 50°C, with high crossflow velocity (6 – 9 m/s-1) and low ΔP (< 0.5 bar). Either the UTP system or ceramic membrane with linear resistance gradient is used.
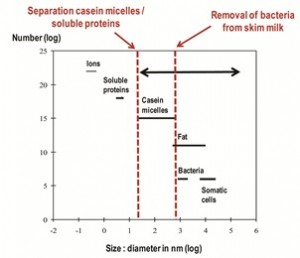
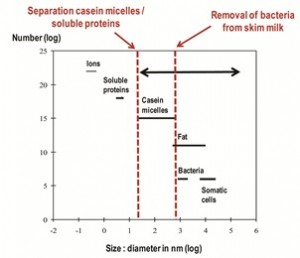
Figure 1 Approximate particle sizes of milk constituents for which separation by means of membrane filtration
can be applied
Milk MF offers several advantages: Permea – tion fluxes are high (400-600 L h-1 m-2) for 10 hours with low casein retention (< one per cent) and high bacteria removal (decimal reduction > 3.5). Spore forming bacteria that represent the main surviving species to pasteurisation are highly retained, so as somatic cells, that range in size from 5 to 30 μm and are able to release numerous thermo-resistant enzymes into the milk with potential impacts on the quality of the products. MF also retains residual fat which is known to strongly influence the efficiency of downstream processes and the resulting properties of dairy products. This process has been commercialised for both drinking and cheese milks.


Figure 2 Evolution of permeation flux, J as a function of
transmembrane pressure, ΔP (pressure-dependent
region in grey)
The microfiltered milks intended for the drinking milk market, encountered great commercial success because of improvement in flavour and storage ability. France is the only country that has officially allowed the comm – ercialisation of microfiltered (also called extended shelf life) raw milk. The skimmed milk is mixed with the amount of heated cream (95°C – 20 seconds) requested for fat standardisation; the mixture is homogenised and aseptically filled. The authorised shelf life at 4-6°C is three weeks. In most countries, to meet current regulatory requirements, microfiltered milk produced using MF and intended for the drinking milk market, undergoes a final pasteurisation step, leading to a claimed shelf life up to five weeks.
In some plants, use of 1.4 μm has been extended as a pretreatment in the production of UHT (Ultra-High-Temperature) milk in order to decrease the intensity of heat treatment with consequently a less cooked taste and an improved storage capability. MF is also used to control the bacteriological quality of the cheese milk. It is claimed that cheeses made from 1.4 μm MF skimmed milk added with past – eurised cream are at least as safe from a hygienic point of view as cheeses made from pasteurised milk. Some cheeses are currently produced by MF, especially those using raw milk: Camembert for instance can be produced from microfiltered milk without significant difference in flavour and texture compared to traditional cheese, provided the adequate microbial ecosystem is added.
MF 1.4 μm of skimmed milk is largely developed in the dairy industry. But due to the high quality of the microfiltered products, MF pretreatment could be expanded to all skimmed milk used for the production of milk derivates such as low-heat milk powder, milk protein concentrates or micellar powders. For instance, MF of skimmed milk could avoid some problems in the use of Milk Protein Concentrates (MPC), such as unacceptable proteolytic activity, caused by a high microbial count originated by growth during UF in spiral-wound equipment. It could also reduce the intensity of the heat treatment before spray-drying and conse quently limits protein denaturation.
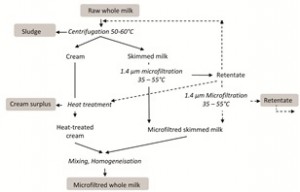
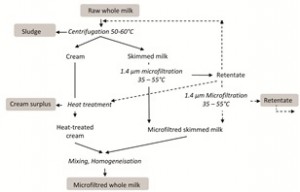
Figure 3 Schematic representation of process for microfiltration of skimmed milk. Dotted lines represent options for
the treatment of retentate
Separation of casein micelles / soluble proteins
For more than 15 years now, MF using a 0.1 μm mean pore diameter membrane has enjoyed rapid industrial development. This operation makes it possible, in one single operation, to separate milk into a retentate enriched specific – ally in native casein micelles (size ~100 – 150 nanometres), and a permeate containing native soluble proteins (size 2 – 10 nanometres)
The content of the retentate is similar to the treated milk but with an increase content in native micellar casein, and consequently a higher content in dry matter, total nitrogen matter and colloidal calcium. This operation has encountered fast-growing success in many plants for making numerous cheese varieties because the retentate is used for the casein enrichment of cheese milk to improve the rennet coagulability of casein and cheesemaking process.
Simultaneously, a crystal clear permeate is obtained. This permeate is often called ‘ideal whey’ because its composition is close to that of a sweet whey but free of rennet-by-productglycomacropeptide, residual fat and microorganisms. Its pH is similar to the milk one, and then higher than all wheys pH that are more acid. It contains lactose, minerals and soluble proteins in their native state, if the previous milk was not heat treated. This permeate is used in the cheese-making process by reincorporation in the casein enriched fraction after denatura – tion under a moderate concentration and a heat treatment. However, due to its composition and because of the native state of its proteins, it is a useful fluid to prepare whey protein concentrates and isolates with high nutritional and functional properties.
At industrial scale, this operation has classically been conducted using ceramic membranes, at 50°C, using the UTP system. At a crossflow velocity of 7 m/s-1, ΔP of 0.5 bar and concentration factor of 2-3, performances are satisfactory: J=75 L h-1m-2 and transmission of soluble proteins is high (up to 80 per cent). Despite that, organic membranes plants have recently been developed in order to reduce costs. With this configuration, system per – formances are low due to the fact that organic membrane plants cannot be conducted using the UTP system and that low temperatures are generally chosen in order to reduce the micro – bial growth during processing. Permeation fluxes are around 10 L h-1m-2 and soluble protein transmission not higher than 45 per cent.
Potential applications
Numerous applications of MF have currently been investigated in the dairy sector. Among them, some are relatively old ideas of fractiona – tion processes, modified and optimised by taking into account the advantages of the recent skimmed milk MF operations. Others are new processes aiming at innovating and creating new products with targeted functionalities.
Based on fractionation processes suggested in the 80s or 90s from cheese wheys, milk MF can be used to prepare a clear permeate enriched in soluble proteins in order to separate β-lactalbumin from previously aggregated α-lactalbumin. The native casein micelle retentate (obtained from milk MF) can also be exploited to extract the C-terminal part of the κ-casein (CMP, caseino-macropeptide), that is used as nutritional protein supplement for people suffering from phenylketonuria. After rennet addition, the CMP is released in the aqueous phase and is separated from the coagulum using MF 0.1 μm. MF can also be used to extract β-casein by exploiting the temperature-dependent association charact – eristics of this protein. When milk is cooled at 4°C, β-casein which is a very hydrophibic protein dissociates from the casein micelles and can be recovered by MF. MF could then still be used to produce numerous bioactive peptides, such as peptides obtained after hydrolysis of β-casein with trypsin.
Among new processes, MF was recently proposed to fractionate the fat globules according to their size. The fractionation of native fat globule is industrially attractive because the native fat globule membrane has very interesting functional and health properties, mainly attributed to its content in phospholipids5,6. It is actually claimed that the use of small globule fraction in cheese prod – uction yields smoother and finer texture, probably because of the interaction of fat globule membrane with the cheese casein matrix, and the differences in triglycerides content of the fat globules according to their size. Moreover, it was recently shown that the lower the fat globule, the higher the enzymatic accessibility, and the better the hydrolysis of small fat globules by both gastric and pancreatic enzymes7.
Other new MF processes are also dedicated to the clarification and re-use of technical fluids such as brines, cleaning solutions in order to reduce the consumption of chemical fluids energy and water, and simultaneously decrease the volume and load of effluents discharged to the waste water purification plant8. Extraction of phospholipids from buttermilk (co-product of the production of butter) using MF is also under investigation.
Conclusions
Microfiltration of skimmed milk using 1.4 or 0.1 μm mean pore diameter membranes are the two main applications of MF in the dairy industry. These technologies are mainly comm – ercialised today for drinking and cheese milks. But in the near future, MF is going to open new horizon for the fractionation of numerous milk components, especially proteins because this operation makes it possible to get fractions with low bacteria and fat content and limited heat treatments, which are always detrimental to the intrinsic biological properties of most of proteins. Moreover, MF operations have gained increasing attention because, in the contact of process sustainability, the wide range of available pore size of MF membrane makes it possible to explore new applications in terms of treatment and recycling of co-products and technical fluids.
References
1. Sandblöm R.M. 1974. Filtering process. Swedish Patent 7416257
2. Garcera D., Toujas A. 1998. Macroporous support with permeability gradient and its manufacturing process, European Patent 08770534 A1 FR 9704359 1-11
3. Skrzypek, M. and M. Burger. 2010. Isoflux ® ceramic membranes – Practical experiences in dairy industry. Desalination 250:1095-1100
4. Gésan-Guiziou G. 2010. Removal of bacteria, spores and somatic cells by centrifugation and microfiltration techniques, in: Griffiths M. “Improving the safety and quality of milk”, Woodhead Publishing Ltd, Cambridge (UK)
5. Goudédranche H., Fauquant J., Maubois J.L. 2000. Fractionation of globular milk fat by membrane microfiltration. Lait, 80: 93-98
6. Michalski M. C., Leconte N., Briard-Bion V., Fauquant J ., Maubois J. L., Goudédranche H. 2006. Microfiltration of raw whole milk to select fractions with different fat globule size distributions: Process optimization and analysis, J. Dairy Sci. 89 (10): 3778-3790
7. Berton, A., Rouvellac B., Robert B., Rousseau F., Lopez C. Crenon I. 2012. Effect of the size and interface composition of milk fat globules on their in vitro digestion by the human pancreatic lipase: native versus homogenized milk fat globules. Food hydrocolloids 29(1): 123-134
8. Alvarez N., Gésan-Guiziou G., Daufin G. 2007. The role of surface tension of reused NaOH on the cleaning efficiency in dairy plants. Int Dairy J. 17:404-411
Biography
Geneviève Gésan-Guiziou is a Research Director at the French Institute for Agricultural Research, INRA. She is currently leading the Dairy Processing team at the STLO research unit (Science and Technology of milk and egg) of INRA, Rennes. Her group mainly works on concentration and fractionation processes. She has co-authored more than 45 papers and 10 book chapters on filtration processes applied to fractionation of dairy components.