Setting the standards for seaweed analysis
- Like
- Digg
- Del
- Tumblr
- VKontakte
- Buffer
- Love This
- Odnoklassniki
- Meneame
- Blogger
- Amazon
- Yahoo Mail
- Gmail
- AOL
- Newsvine
- HackerNews
- Evernote
- MySpace
- Mail.ru
- Viadeo
- Line
- Comments
- Yummly
- SMS
- Viber
- Telegram
- Subscribe
- Skype
- Facebook Messenger
- Kakao
- LiveJournal
- Yammer
- Edgar
- Fintel
- Mix
- Instapaper
- Copy Link
Posted: 5 March 2021 | Jens J Sloth, Susan L Holdt | No comments yet
With growing consumer demand for seaweed in European countries, Jens J Sloth and Susan L Holdt of the Technical University of Denmark explain why the lack of seaweed regulation is an immediate concern, and how laboratories should prepare for new legislation.
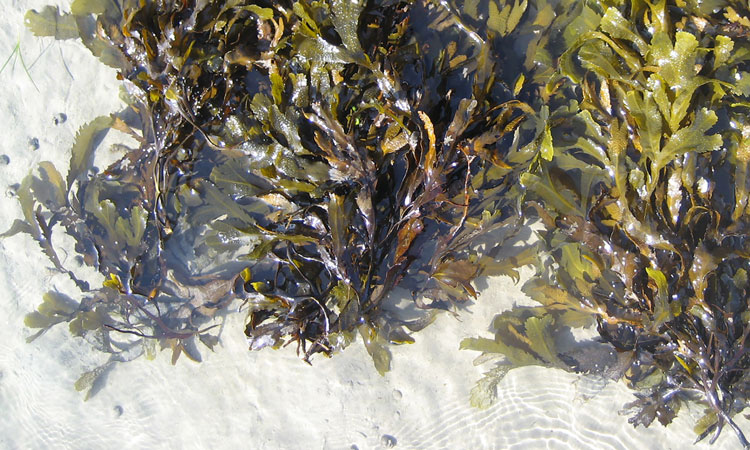
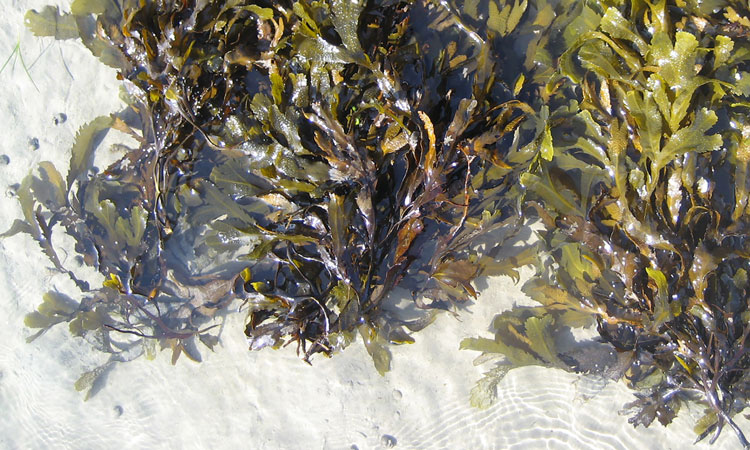
From traditional sushi to afternoon snacks, seaweed – a staple food in Asia – has experienced a significant rise in consumption in European countries over the past decade. The recent shift in western diets towards more plant-based and sustainable food sources is poised to further boost this trend. It is anticipated that by 2050, 0.1 percent of our oceans could be dedicated to producing seaweed as a food source, generating 15 times more seaweed than current production in order to meet increasing global demand.1
From traditional sushi to afternoon snacks, seaweed – a staple food in Asia – has experienced a significant rise in consumption in European countries over the past decade. The recent shift in western diets towards more plant-based and sustainable food sources is poised to further boost this trend. It is anticipated that by 2050, 0.1 percent of our oceans could be dedicated to producing seaweed as a food source, generating 15 times more seaweed than current production in order to meet increasing global demand.1
Despite the recent explosive growth of seaweed as a food product, there are currently no food regulations driving the safety and quality of seaweed outside of Asia, posing a potential threat to consumer health. Here, we discuss why there is a pressing need to establish and enforce regulatory standards for seaweed, and what laboratories can do to better prepare for the impending change in requirements.
Standardisation in the seaweed domain
Seaweed is popularly seen in the western world as a ‘superfood’, associated with healthier lifestyles and sustainability. It’s high nutritional value comes from macro- and micro-nutrients, such as vitamin B12, dietary fibres and omega-3 fatty acids, among others, and it serves as a rich source of other bioactive compounds (eg, polyphenols, sulphated polysaccharides, pigments) that carry health benefits.
Due to their characteristic cell wall structure possessing high biosorption properties, seaweeds accumulate minerals and essential trace elements from their immediate environment. However, this high accumulation potential may also lead to the seaweed amassing potentially harmful elements found in its surrounding waters. As a result, toxic elements, such as cadmium, lead, mercury, inorganic arsenic and iodine, to name a few, are typically found in seaweeds at levels that are several orders of magnitude higher than in the water.2 Unregulated distribution of seaweed species carrying these elements at high concentrations may lead to detrimental health effects on the unsuspecting consumer.
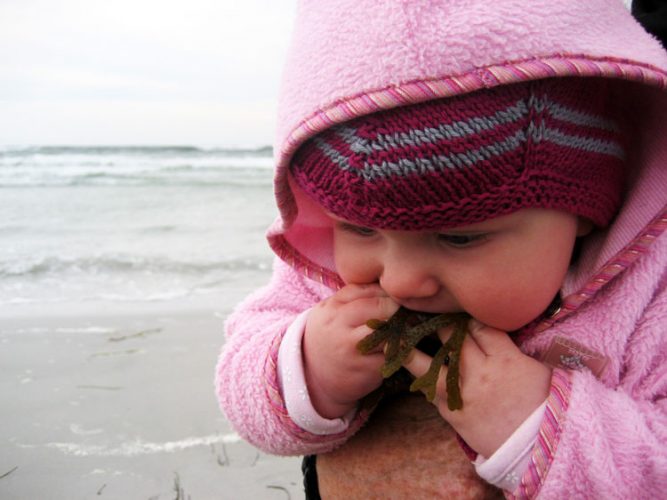
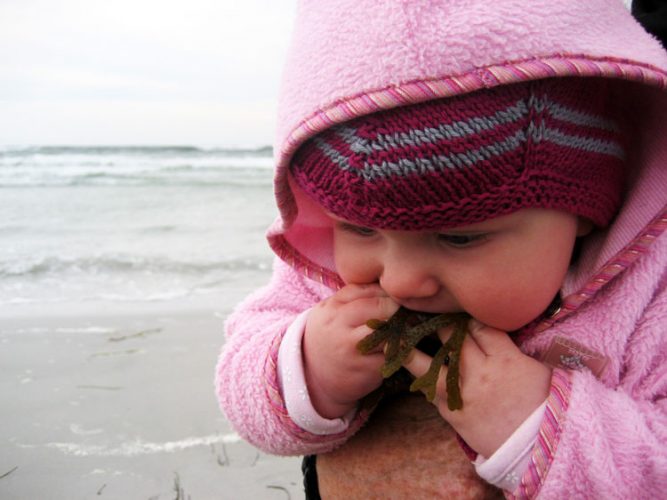
People of all ages enjoy eating seaweed
Certain species of seaweed can contain extremely high amounts of iodine – up to 10 g/kg dry weight3 – resulting in excessive iodine levels when consumed in even small amounts. Although an essential micronutrient for thyroid hormone synthesis, exposure to higher levels of iodine can trigger thyroid gland dysfunction. In fact, based on the European Food Safety Authority (EFSA) recommendations, the upper level for iodine intake is set at 600μg per day for adults,4 making iodine levels in seaweed a potential health concern. Mercury, lead, cadmium and inorganic arsenic, on the other hand, have no biological role in the human body and can be harmful even when consumed in trace amounts. Moreover, as lead is classified as possibly carcinogenic and neurotoxic, and inorganic arsenic is classified as a “class I carcinogen”,5 their presence in seaweed puts the health of consumers at serious risk.
It must also be considered that different seaweed species show different biosorption preferences for these toxic elements. In addition, other external variables, such as geographic location, aquatic environment, season, sampling or processing methods, can further modulate the elemental composition, sometimes even within the same seaweed species.
The potential toxicological profile of seaweed has caught the attention of several food authorities concerned about consumer health and safety related to seaweed consumption. Currently, there are no harmonised EU regulations on the maximum allowable levels of these elements in seaweed being consumed as food, despite its growing popularity. However, emerging interest in the use of seaweed in food and feed application, coupled with insufficient data on contaminant levels, has spurred a long-awaited conversation on harmonising seaweed regulations and standards. As a result, in 2018, the European Commission advised EU member states to monitor the presence of elements in seaweeds.6 The results will subsequently be compiled by EFSA, which will use occurrence and consumption data to estimate the exposure to harmful elements from seaweed consumption and, based on this, advise the EU Commission on potential subsequent regulatory measures to be taken; ie, the establishment of maximum levels.
Monitoring samples to set new standards
In recent years, our team at the National Food Institute, Technical University of Denmark has been involved in several research projects, with the aim of monitoring elemental composition for the most common edible species of seaweeds. We have quantified the levels of lead, mercury, inorganic arsenic, cadmium and iodine found in seaweeds – mainly seaweeds harvested from natural populations and cultivated seaweeds from Denmark, but also samples from collaborators in other countries.
In one of the projects, seven different seaweed species from Danish waters were sampled; namely, Fucus vesiculosus, Fucus serratus, Fucus spiralis, Fucus evanescens, Saccharina latissima, Ulva lactuca and Cladophora sp. Iodine was found at the highest levels in all the samples studied, followed by total arsenic and lead.7 It is of note that total arsenic values may not be directly applicable to risk assessment studies as arsenic may be present as part of an organic molecule, exhibiting a potentially lower toxicity. However, these numbers provide an important starting point for measuring levels of inorganic arsenic, the more toxicologically relevant species.
Upon obtaining these levels, we then performed a risk assessment of dietary exposure to iodine, cadmium, lead and mercury in our seaweed samples. Due to the lack of seaweed consumption data for Europeans, we hypothesised a single serving size of 5g of seaweed, once a week. Our assessment found that the intake of iodine in all seven species can easily exceed the prescribed EFSA daily intake limits.7 This is especially harmful to high-risk subgroups, such as pregnant women, children and individuals with thyroid dysfunction.
Although the EU provides recommendations on the maximum intake levels of individual elements, such as arsenic, cadmium, lead and iodine, their direct presence in seaweed consumed as food isn’t regulated or documented. Using our newly available data on seaweed elemental composition, alongside other data stemming from multi-institute collaborations, regulators can make science-based decisions and pass new legislation. To meet new regulatory guidelines, companies that currently use data from scientific literature to create food labels will soon need to perform their own trace analysis to ensure regulatory compliance. As such, the food community as a whole will need standardised methods to analyse seaweed.
Analytical methods for seaweed analysis
Several European laboratories have adopted inductively coupled plasma mass spectrometry (ICP-MS) as the analytical method of choice for trace elemental quantification in seaweeds. The ICP ionisation source is particularly well-suited to decompose the sample into constituent elements – a necessary step for elemental analysis – and then transform them into ions for subsequent MS quantitation. With the capacity to detect multiple elements in a single run, down to nanogram levels per litre, ICP-MS protocols for elements such as cadmium, lead, arsenic, iodine and mercury have already been standardised by the European Committee for Standardisation (CEN) for other applications.8 These available protocols can now be validated for seaweed-specific applications once sample preparation steps have been optimised.
Given the concentration profiles of the trace elements found in seaweed species, laboratories must ensure that the ICP-MS platform chosen, along with the accompanying experimental protocol, meets the following key requirements:
- Offers high sensitivity to measure trace levels of analytes
- Provides a high linear range to examine a spectrum of concentration levels in a single run
- Includes optimised dilution and rinsing protocols, especially when dealing with high iodine concentrations that can contaminate the instrument
- Assures complete removal of all spectral interferences, eliminating potentially false positive results on key analytes.
Using modern analytical systems ensures that the method is sensitive, linear and reproducible, especially for method development and validation that will set the foundation for future experiments. In addition, coupling ICP-MS to ion chromatography (IC) separation allows elemental speciation analysis. For instance, laboratories may employ anion‑exchange IC to separate inorganic arsenic from its less toxic organic form to obtain a better picture of the safety profile of the samples.
IC coupling with ICP-MS can also be used to distinguish between the different chemical forms of iodine for more detailed analysis. Knowing the types of iodine species present in seaweed can then be used to develop methods during the processing stage, to reduce iodine content in the final product.
In a recent study performed by our researchers at DTU Food, the hyphenation of IC and ICP-MS resulted in detection and quantification of three major iodine species present in seaweed samples – namely, monoiodo-tyrosine (MIT), diiodo-L-tyrosine dehydrate (DIT) and iodide – in only 13 minutes.9
As laboratories validate newer and faster methods for seaweed analysis, the food community moves closer to standardisation. After a reliable method is developed and tested, technological add‑ons, such as autosamplers or liquid handlers, may be added to the workflow for higher throughput routine analyses.
Preparing for regulatory change
Standardising seaweed analysis with enforced government regulation is long overdue in European countries. Our recent collaborative efforts in monitoring seaweed samples, along with other follow-up studies, will provide food authorities with the necessary information to evaluate the need for establishing maximum levels of toxic elements allowed in seaweeds, thus limiting human exposure.
At the brink of new legislation, it’s only a matter of time before laboratories are required to perform seaweed analysis, irrespective of its intended use. Preparing for this change will involve updating in-house technologies to detect trace elements and their species. As seaweed regulations continue to evolve and become more stringent, choosing modern analytical systems will ensure laboratories are future-proofed against changing requirements.
References
1. Giercksky E, Doumeizel V. 2020, Seaweed Revolution: A Manifesto for Sustainable Future. Accessed 1st December 2020: https://ungc-communications-assets.s3.amazonaws.com/docs/publications/The-Seaweed-Manifesto.pdf
2. Jadeja RN, Batty L. 2013. Metal content of seaweeds in the vicinity of acid mine drainage in Amlwch, North Walwa, U.K. Indian Journal of Geo-Marine Sciences, 42, 16–20
3. Duinker A, Roiha IS, Amlund H, et al. 2016. Potential risks posed by macroalgae for application as feed and food – a Norwegian perspective, National Institute of Nutrition and Seafood research. DOI: 10.13140/RG.2.2.27781.55524
4. Tolerable upper intake levels for vitamins and minerals, 2006. European Food Safety Authority. Accessed 1st December 2020: https://www.efsa.europa.eu/sites/default/files/efsa_rep/blobserver_assets/ndatolerableuil.pdf
5. Arsenic and Cancer Risk, American Cancer Society. Accessed 1st December 2020: https://www.cancer.org/cancer/cancer-causes/arsenic.html#:~:text=The%20EPA%20classifies%20inorganic%20arsenic,%2C%20skin%2C%20and%20liver%20cancers.
6. Commission Recommendation (EU) 2018/464 of 19 March 2018 on the monitoring of metals and iodine in seaweed, halophytes and products based on seaweed, C/2018/1560. Accessed 1st December 2020: https://eur-lex.europa.eu/legal-content/EN/TXT/?uri=uriserv:OJ.L_.2018.078.01.0016.01.ENG&toc=OJ:L:2018:078:TOC
7. National Food Institute, Technical University of Denmark, Denmark, Sa Monteiro
8. Monteiro MS, Sloth J, Holdt S, Hansen M. 2019. Analysis and Risk Assessment of Seaweed. EFSA Journal
9. 2019;17(S2):e170915, 11 pp. https://doi.org/10.2903/j.efsa.2019.e170915
10. CEN/TC 275/WG 10 – Elements and their chemical species. Accessed 1st December 2020: https://standards.cen.eu/dyn/www/f?p=CENWEB:7:0::::FSP_ORG_ID:7909&cs=1F990598785D4F3CE15198479C4015BDF
11. Jerše A, Sloth JJ, Kutscher D, Fisher C. 2020. Determination of iodine containing species in seaweed using IC-ICP-MS
Jens is a professor in analytical food chemistry at the National Food Institute at the Technical University of Denmark. He is research group leader of The Research Group for Analytical Food Chemistry, which works with development and application of analytical methods for determination of various chemical compounds in food, feed and biological samples. Jen has extensive experience within various research projects on toxic and essential trace elements in food and feed, with special emphasis on the use of ICP-MS as an analytical tool box. For more than a decade, he has been an expert member of the CEN standardisation committees for trace elements in food and feed and contributed to the development of new standardised methods of analysis for the determination of trace elements and their species. In 2018, he was appointed as director of the European Reference Laboratory for metals and nitrogenous compounds in feed and food (EURL-MN).
Susan is a biologist and associate professor at DTU Food. Her field of research is within seaweed composition, bioactives and technologies for extraction. She is also involved in research on seaweed as a food, legislation, standardisation, retaining the qualities of seaweed, and reducing the contaminants found in seaweed.
Issue
Related topics
Food Safety, Health & Nutrition, Quality analysis & quality control (QA/QC), Regulation & Legislation