Securing the fermentation of yoghurts against spore-forming pathogens
- Like
- Digg
- Del
- Tumblr
- VKontakte
- Buffer
- Love This
- Odnoklassniki
- Meneame
- Blogger
- Amazon
- Yahoo Mail
- Gmail
- AOL
- Newsvine
- HackerNews
- Evernote
- MySpace
- Mail.ru
- Viadeo
- Line
- Comments
- Yummly
- SMS
- Viber
- Telegram
- Subscribe
- Skype
- Facebook Messenger
- Kakao
- LiveJournal
- Yammer
- Edgar
- Fintel
- Mix
- Instapaper
- Copy Link
Posted: 5 December 2024 | Jean-Christophe AUGUSTIN, John BASSETT, Rosalia TRIAS MANSILLA, Stéphane DAGNAS | No comments yet
Colleagues from the Danone Food Safety Center in France reveal the method for ensuring the safe fermentation of dairy and plant-based yoghurts and preventing toxin formation.
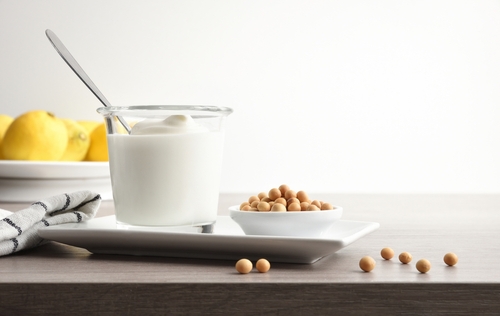
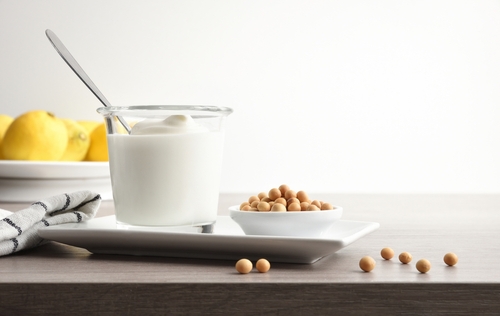
Dairy and plant-based yoghurts are obtained via lactic fermentation of pasteurised raw materials where bacterial spores of Bacillus cereus and Clostridium botulinum can still be present. Conditions during the initial phase of the fermentation favour outgrowth of these spores, potentially resulting in toxin formation. This risk is controlled by ensuring an inhibitory pH of 5.5 is achieved in a certain time frame during the fermentation. This ‘pH 5.5 rule’ has historically been effective in managing the risk linked to spore formers for fast-acidifying dairy fermentations, but predictive models are of primary importance to assess innovative fermentations with alternative carbohydrate sources or longer fermentation times.
Microbiological issues
The quality and safety of fermented dairy products, and more recently of plant-based alternatives, is conventionally ensured by:
i) pasteurisation, eliminating heat-sensitive pathogens potentially present in raw materials (vegetative bacteria, viruses, protozoa)
ii) the addition of lactic acid bacteria fermenting the milk, whose growth leads to acidification, controlling the germination and outgrowth of heat-resistant spores (eg, Bacillus, Clostridium)
iii) refrigeration of finished products, which is also essential for quality, as it limits the development of acid-tolerant spoilage organisms such as yeasts and moulds (Figure 1).
The critical step for food safety is the beginning of fermentation when conditions are favourable to toxin production by spore-forming pathogens like B. cereus and C. botulinum (Figure 1). Temperature is optimal, typically between 35°C and 42°C, and the pH of milk or plant-based slurries is near neutral since the acidification by lactic acid bacteria has not yet started.
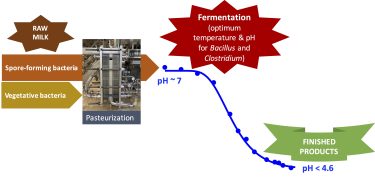
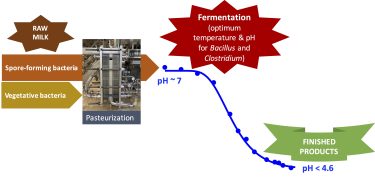
Figure 1: Technological steps of fermented products (yoghurt type), associated microbiological risks, control measures as well as critical step (start of fermentation).
B. cereus can grow at the early stages of fermentation but the viable population reduces when pH is lowered at the end of fermentation.1 The B. cereus population is therefore usually low in yoghurts but in the case of emetic B. cereus strains, cereulide toxin may be produced in the initial stage of fermentation, making yoghurt unsafe. The production of cereulide is inhibited by fast-acidifying starter cultures but its growth is possible with slow-acidifying fermentations. The potential for production of cereulide by mesophilic emetic strains has been demonstrated internally with dairy yoghurt and also recently confirmed with plant-based fermented products.2 C. botulinum multiplication and botulinum neurotoxins formation are generally not expected in yoghurt3 and yogurt-type products have rarely been implicated in botulism outbreaks.4-6 In some cases, the toxin clearly came from external sources,6 but for others, it is unclear whether or not the toxin was produced during fermentation.4,5 It is well known that incomplete fermentation with too slow or insufficient reduction in pH can create conditions that support toxin production by C. botulinum.7 Therefore, pH monitoring is important to control the risk linked to spore formers during yoghurt fermentation.
Experiments carried out at Danone in the late 1990s confirmed that B. cereus can grow when dairy fermentation starts but is inhibited when pH approaches approximately 5.5 (Figure 2). The time to reach pH 5.5 is then used as an operational parameter in Danone factories as the ‘pH 5.5 rule’ to control the risk linked to B. cereus. Maximum times allowed to reach pH 5.5 have been estimated to keep the B. cereus population below the safety threshold, ie, 5 log10CFU/g,8 accounting for the initial B. cereus load. Figure 2 illustrates that growth stops after approximately four hours when the pH decreases to 5.5. There is approximately a 2-log increase in the B. cereus population before reaching pH 5.5 – this fermentation is therefore considered safe if the initial B. cereus contamination is below 3 log10CFU/g in order not to exceed 5 log10CFU/g during the fermentation.
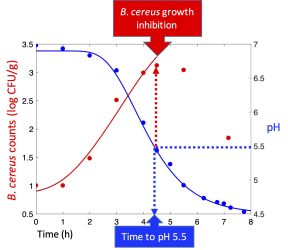
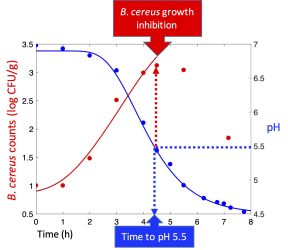
Figure 2: Evolution of mesophilic B. cereus during yogurt fermentation. Red circles are observed counts and the red line is the predicted growth of B. cereus according to the pH (blue line and circles).
Even if low pH is the primary factor in the preservation of lactic acid-fermented foods, other factors like lactic acid concentration and its direct antimicrobial effect could also be significant; this is probably the case here, since the minimum pH for growth of B. cereus is lower than 5.5, typically 4.6-4.7,2,3 when other environmental factors are optimum (ie, in microbiological-rich medium at optimum temperature for growth). pH monitoring and the ‘pH 5.5 rule’, based on these empirical observations, are historically effective to manage the risk linked to spore formers for fast-acidifying dairy fermentations (ie, fermentation times below 10 hours). However, refined models are needed to describe the behaviour of Bacillus and Clostridium to secure new fermentations with alternative carbohydrate sources and/or longer fermentation times.
Modelling the behaviour of B. cereus during yoghurt fermentation
The growth of B. cereus during fermentation of dairy and plant-based yoghurts can be predicted using mathematical models that describe:
the evolution of pH during the yoghurt fermentation and the lactic acid produced according to their specific composition and buffering property (Figure 3) and the behaviour of B. cereus.
The microbial behaviour is predicted using cardinal predictive models9 according to the pH and the inhibitory effect of the undissociated form of lactic acid. These models seem appropriate for this purpose (Figure 4).
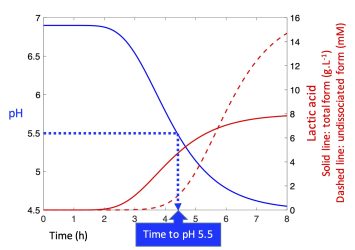
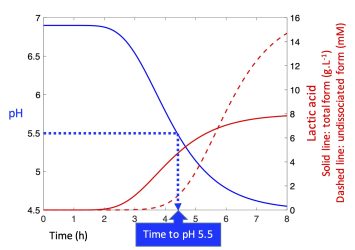
Figure 3: Example of evolution of the pH and lactic acid content during yogurt fermentation.
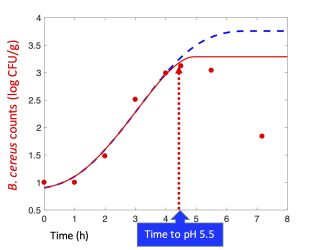
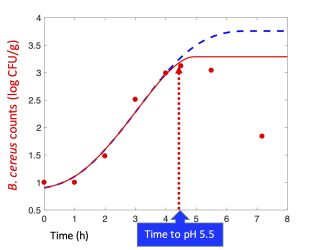
Figure 4: Predicted growth of mesophilic B. cereus during yoghurt fermentation accounting for the pH evolution (dashed blue line) and the combined effect of pH and lactic acid (solid red line).
Predicted behaviour of C. botulinum during yoghurt fermentation
A similar approach is used to predict the behaviour of proteolytic mesophilic strains as well as non-proteolytic psychrotrophic strains of C. botulinum during yoghurt fermentation (Figure 5). A halt in growth is predicted for both types when the pH reaches approximately 5.5. Therefore, the ‘pH 5.5 rule’ also seems effective to manage the botulinum risk. This is not surprising, as the minimum pH and minimum inhibitory concentrations for lactic acid of B. cereus and C. botulinum are close. The minimum pH for proteolytic C. botulinum is 4.6 and 5.0 for non-proteolytic C. botulinum,10 which explains the faster inhibition of non-proteolytic strains compared to proteolytic strains (Figure 5).
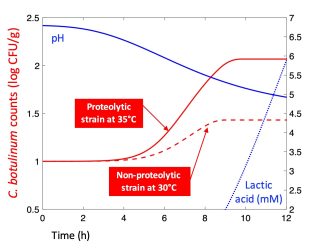
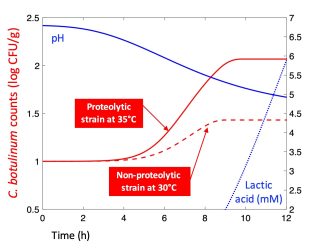
Figure 5: Predicted growth of C. botulinum during yogurt fermentation taking into account the combined effect of pH and lactic acid.
Controlling the risk linked to spore formers during the fermentation of yogurt
Models help to determine the maximum time to reach pH 5.5 with the objective to maintain the B. cereus population below the safety threshold of 5 log10CFU/g and to limit the growth of C. botulinum to an acceptable level. Since botulinum neurotoxins synthesis occurs during the transition from the exponential to the stationary growth phase8,11 and considering that a 2.2-mean log has been deemed necessary to observe toxin formation for non-proteolytic C. botulinum12 and a 2-log increase is needed for proteolytic strains,13,14 a safety threshold increase for C. botulinum of 1 log10CFU/g can be proposed.
In most cases, the maximum time to reach pH 5.5 is determined by B. cereus when its initial load is high, ie, 2-3 log10CFU/g, or when the fermentation temperature is above 35°C. But when the temperature is below 30°C, the time limit is dependent on non-proteolytic C. botulinum and dependent on proteolytic C. botulinum for intermediate temperatures between 30 and 35°C. For long fermentations where the ‘pH 5.5 rule’ cannot be respected, the pasteurisation of raw materials must be replaced by a sterilisation to inactivate bacterial spores before the fermentation step. The predictive models reinforce the validity of the ‘pH 5.5 rule’ and can help to define the maximum time allowed to reach pH 5.5 during fermentation while limiting growth of contaminants like B. cereus and C. botulinum, thus keeping finished products safe.
AUTHORS
Jean-Christophe AUGUSTIN, Stéphane DAGNAS, Rosalia TRIAS MANSILLA, John BASSETT
Danone Food Safety Center, RD128, F-91190 Gif-Sur-Yvette, France
REFERENCES
- Røssland E, Andersen Borge GI, Langsrud T, Sørhaug T. 2003. Inhibition of Bacillus cereus by strains of Lactobacillus and Lactococcus in milk. J. Food Microbiol. 89:205-12.
- Kyrylenko A, Beekmann K, Mahajan I, et al. 2024. Risks of emetic Bacillus cereus outgrowth in plant-based fermentations. IAFP Europe, Geneva 2024.
- Lindström M, Myllykoski J, Sivelä S, Korkeala H. 2010. Clostridium botulinum in cattle and dairy products. Rev. Food Sci. Nutr. 50:281-304.
- Akdeniz H, Buzgan T, Tekin M, et al. 2007. An outbreak of botulism in a family in Eastern Anatolia associated with eating süzme yoghurt buried under soil. J. Infect. Dis. 39:108-14.
- Boostani R, Olfati N, Taheri NS, et al. 2021. An outbreak of food-borne botulism caused by a traditional yogurt. Asia Pacific J. Medical Toxicol. 10:113-116.
- O’Mahony M, Mitchell E, Gilbert RJ, et al. 1990. An outbreak of foodborne botulism associated with contaminated hazelnut yoghurt. Infect. 104:389-395.
- Austin JW. 2003. Clostridium: Occurrence of Clostridium botulinum, p. 1407-1413. In B. Caballero (ed.), Encyclopedia of Food Sciences and Nutrition (Second Edition), Academic Press.
- Bradshaw M, Dineen SS, Maks ND, Johnson EA. 2004. Regulation of neurotoxin complex expression in Clostridium botulinum strains 62A, Hall A-hyper, and NCTC 2916. Anaerobe. 10:321-33.
- Le Marc Y, Baert L, Buss da Silva N, et al. 2021. The effect of pH on the growth rate of Bacillus cereus sensu lato: Quantifying strain variability and modelling the combined effects of temperature and pH. J. Food Microbiol. 360:109420.
- EFSA Panel on Biological Hazards (BIOHAZ). 2012. Scientific Opinion on public health risks represented by certain composite products containing food of animal origin. EFSA Journal 10:2662.
- Couesnon A, Raffestin S Popoff MR. 2006. Expression of botulinum neurotoxins A and E, and associated non-toxin genes, during the transition phase and stability at high temperature: analysis by quantitative reverse transcription-PCR. Microbiology 152:759-770.
- Koukou I, Mejlholm O, Dalgaard P. 2021. Cardinal parameter growth and growth boundary model for non-proteolytic Clostridium botulinum – Effect of eight environmental factors. J. Food Microbiol. 346:109162.
- Ter Steeg PF, Cuppers HGAM, Hellemons JC, Rijke G. 1995. Growth of proteolytic Clostridium botulinum in process cheese products: I. Data acquisition for modeling the influence of pH, sodium chloride, emulsifying salts, fat dry basis, and temperature. Food Prot. 58:1091-1099.
- Ward S, Golden M, Wanless BJ, et al. 2023. Correlating Clostridium botulinum growth with botulinum neurotoxin production using the DigELISA in model meat systems. IAFP Annual Meeting, Toronto 2023.