Whole room disinfection – potential for environmental pathogen control?
- Like
- Digg
- Del
- Tumblr
- VKontakte
- Buffer
- Love This
- Odnoklassniki
- Meneame
- Blogger
- Amazon
- Yahoo Mail
- Gmail
- AOL
- Newsvine
- HackerNews
- Evernote
- MySpace
- Mail.ru
- Viadeo
- Line
- Comments
- Yummly
- SMS
- Viber
- Telegram
- Subscribe
- Skype
- Facebook Messenger
- Kakao
- LiveJournal
- Yammer
- Edgar
- Fintel
- Mix
- Instapaper
- Copy Link
Posted: 5 November 2010 | Alicja Malinowska & John Holah, Campden BRI | No comments yet
To meet retailer, customer and consumer expectations, there are increasing demands within the food industry for higher standards of microorganism control in food production environments. Traditional approaches such as cleaning and disinfection regimes have been targeting specific sites within the processing environment to control contamination. Such sites might include food production equipment, where much of the rest of the processing area is not routinely decontaminated.
To sustain day-to-day control of pathogens, this targeted cleaning and disinfection approach is adequate, but does not eliminate all microorganisms. Previous research at Campden BRI has demonstrated that microbial strains, including pathogens, can become persistent in food factories and survive for several years1,2.
To meet retailer, customer and consumer expectations, there are increasing demands within the food industry for higher standards of microorganism control in food production environments. Traditional approaches such as cleaning and disinfection regimes have been targeting specific sites within the processing environment to control contamination. Such sites might include food production equipment, where much of the rest of the processing area is not routinely decontaminated. To sustain day-to-day control of pathogens, this targeted cleaning and disinfection approach is adequate, but does not eliminate all microorganisms. Previous research at Campden BRI has demonstrated that microbial strains, including pathogens, can become persistent in food factories and survive for several years1,2.
To meet retailer, customer and consumer expectations, there are increasing demands within the food industry for higher standards of microorganism control in food production environments. Traditional approaches such as cleaning and disinfection regimes have been targeting specific sites within the processing environment to control contamination. Such sites might include food production equipment, where much of the rest of the processing area is not routinely decontaminated.
To sustain day-to-day control of pathogens, this targeted cleaning and disinfection approach is adequate, but does not eliminate all microorganisms. Previous research at Campden BRI has demonstrated that microbial strains, including pathogens, can become persistent in food factories and survive for several years1,2.
The requirement for further reduction of pathogens and the identification of persistent strains has led to a significant interest in the use of whole room disinfection techniques to supplement routine cleaning and disinfection. In addition to routine cleaning and disinfection, disinfectant aerosols in the form of fogs and mists have traditionally been used in production areas to reduce the number of airborne microorganisms and also to apply disinfectant to surfaces that may be difficult to reach. To provide guidance to the food and drink industry, a Campden BRI member subscription-funded research project was undertaken to compare the efficacy of such chemical fogging with novel ‘whole room’ techniques such as gaseous ozone and hydrogen peroxide vapour (HPV). These gases have the potential to penetrate all exposed environmental surfaces and have thus been described as whole room techniques.
Laboratory trials
Listeria monocytogenes, Pseudomonas aeruginosa and Staphylococcus aureus were selected as test microorganisms because of their known presence, and in some cases persistence, in the food factory environment. Decontamination experiments were carried out in a sealed, aerobiology laboratory (volume 43 m3) where two centimetre diameter, stainless steel discs (Grade 2 B 1.4301), previously sterilised and inoculated with 0.05 millilitres of 108 cfu ml-1 test suspension (without soil), were attached to shelves on five metal stands allocated around the room. The surfaces were positioned in three orientations: horizontally, vertically and underneath the shelf. The room was sealed and the surfaces exposed to each decontamination system for the required period of time. After treatment, microorganisms were recovered from the discs in accordance with BS EN 136973 using nine millilitre diluent (Tryptone, pancreatic digest of casein, Sodium Chloride, Deionised water) containing five grams of sterile glass beads (with a diameter of three to four millimetres) and one millilitre universal disinfectant inactivator. Each sample was serially diluted in diluent to 10-4 and plated out in duplicate using TSA (BS) agar with appropriate controls for bacteria remaining on the discs.
Results
A commercial quaternary ammonium based chemical disinfectant was fogged into the chamber according to the chemical manufacturer’s instructions. A total of 20 stainless steel discs per test microorganism were located around the chamber in different orientations (horizontal, vertical and underneath) and the log reductions obtained are shown in Figure 1. The results achieved are typical for a chemical fog and indicate that decontamination is strongest on horizontal surfaces (particularly at lower levels), on which the majority of the disinfectant aerosols settle.
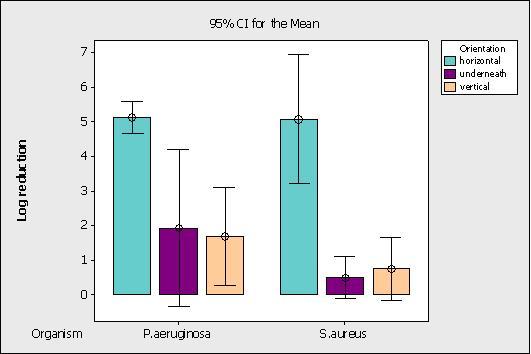
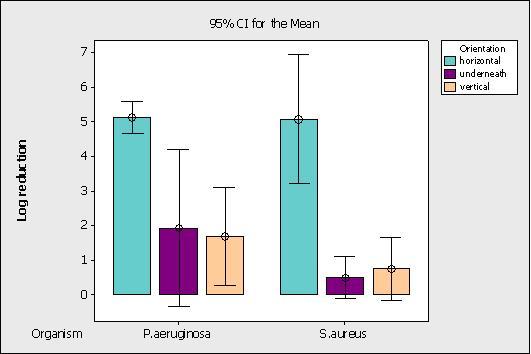
Figure 1 Effect of chemical fogging on microbiological log reduction depending on surface orientation
A good level of disinfection is achieved on horizontal surfaces (5 logs), which is above that required for surface disinfectants as defined by the European Standard surface disinfectant test EN 13697, in which a 4 log reduction is required to pass the standard.
HPV trials were undertaken using the Bioquell Clarus R/R2 unit following the manufacturer’s instructions. Experiments at 10 g/m3 and 20 g/m3 involved the testing of 60 stainless steel discs per microorganism tested whilst experiments at 30 g/m3 and 40 g/m3 involved the testing of six stainless steel discs located around the test chamber. Log reductions are shown in Figure 3. A relatively poor log reduction of approximately 1.5 logs was achieved for P. aeruginosa and S. aureus at 10g/m3, the default concentration for the HPV equipment, though this was substantially increased to an approximate 3 log reduction when this concentration was doubled to 20 g/m3. There was little difference, however, in the log reductions achieved between the vegetative microorganisms tested, with S. aureus being slightly more resistant. When the concentration was increased to 30 and 40 g/m3, different survival rates between the vegetative microorganisms was seen. L. monocytogenes was seen as the least resistant microorganism and its survival was reduced with these increasing concentrations, though there was no significant difference in survival at 30 or 40 g/m3. The survival of P. aeruginosa was also reduced with concentration, though the log reduction at 40 g/m3 was only marginally significantly different than at 20 g/m3. There was no significant difference in the susceptibility of S. aureus with increasing HPV concentration above 20 g/m3.
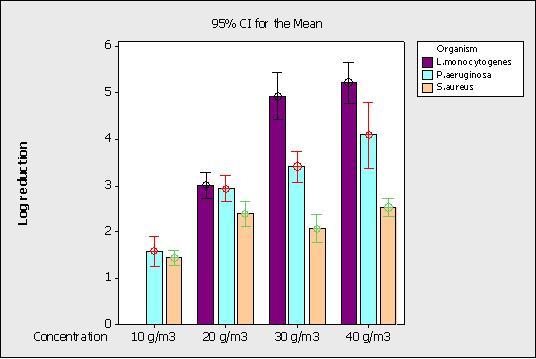
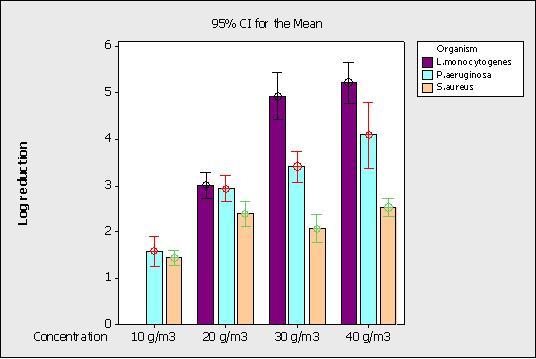
Figure 3 Effect of H2O2 vapour on microbiological log reduction at different concentrations
Figure 4 opposite shows the effect of surface orientation on the decontamination of P. aeruginosa, S. aureus and L. monocytogenes by HPV at 20g/m3. No statistical differences were seen between orientations for all organisms.
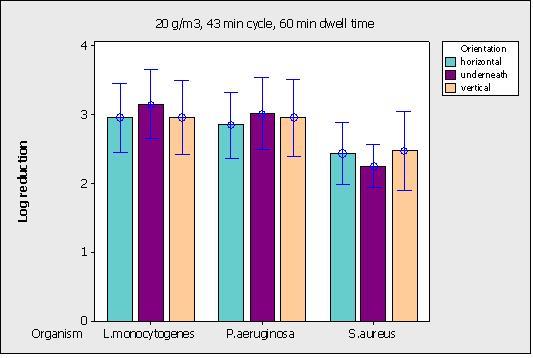
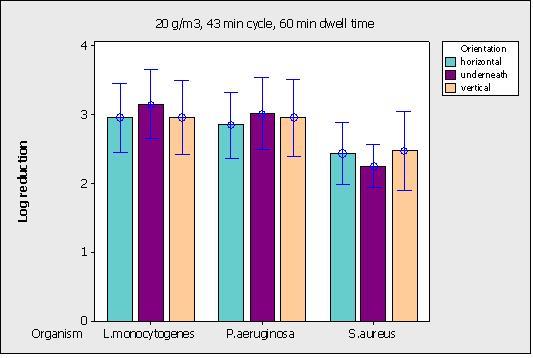
Figure 4 Effect of H2O2 vapour on microbiological log reduction depending on orientation at 20 g/m3
Ozone decontamination trials were undertaken using a Radical Small Mobile Unit (Figure 5) from Steritrox, according to the manufacturer’s instructions. Experiments at 8 ppm for 30 minutes (total cycle 70 minutes), 20 ppm for 30 minutes (total cycle 210 minutes) and 25 ppm for 90 minutes (total cycle 210 minutes) involved the testing of 60 stainless steel discs per microorganism which were located around the testing room in different orientations. Log reductions achieved for the test microorganisms are shown in Figure 6.
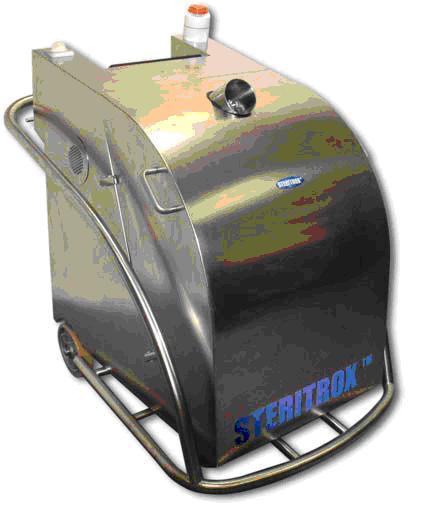
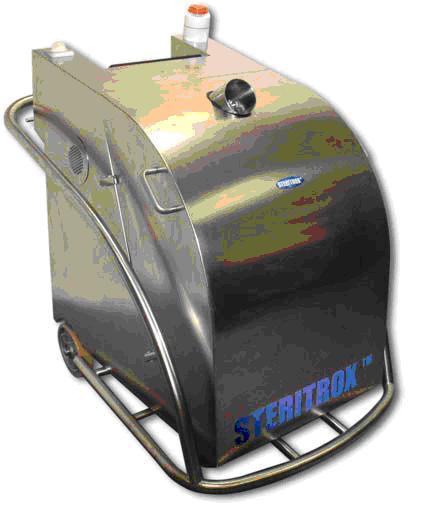
Figure 5The Radical Small Mobile Unit (UMB) from Steritrox
The results in Figure 6 show two trends. Firstly, there is a clear relationship between ozone concentration and log reduction, with the log reduction for S. aureus ranging from 0.7 logs at 8 ppm to 1.5 logs at 20 ppm and 2.1 logs at 25 ppm. Secondly, the effect of ozone on the three vegetative strains tested is markedly different with, at 20 ppm, S. aureus being most resistant, followed by P. aeruginosa, and L. monocytogenes being most sensitive. There was no practical difference in the log reductions achieved for all micro – organisms with orientation.
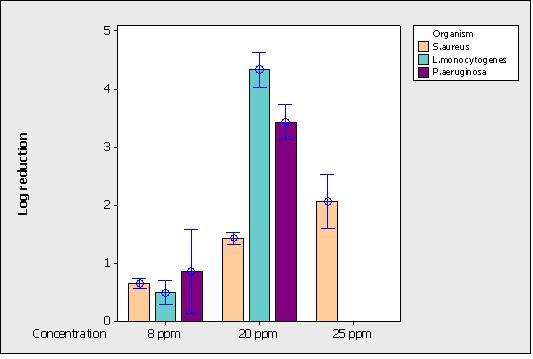
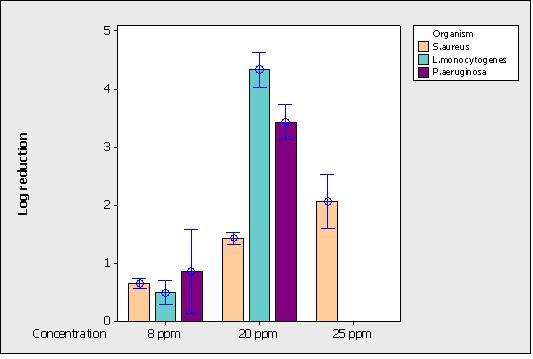
Figure 6 Effect of gaseous O2 on microbiological log reduction at different concentrations
Discussion
The results achieved for chemical fogging are typical for quality disinfectants in that good decontamination is achieved on horizontal surfaces but little is achieved on other surface orientations. These findings are in agreement with those of Campden BRI and the Silsoe Research Institute which undertook a MAFF funded project in 19884. This work suggested that fogging is effective at reducing airborne microbial populations by 2 to 3 log orders in 30 to 60 minutes and horizontal surfaces up to 6 log orders in 60 minutes, with minimal effect on vertical surfaces and underneath equipment. Chemical fogging is therefore useful for the periodic decontamination of the air and as an automated method to apply disinfectants to accessible, food processing equipment surfaces. It will have little or no effect, however, on ceilings and overhead structures.
At low concentrations of HPV (10-20 g/m3), P. aeruginosa, S. aureus and L. monocytogenes (all of which are catalase positive) were all able to partially resist the concentration/volume of disinfectant that they came into contact with. When the HPV concentration was increased to 30 and 40 g/m3, an increase in susceptibility was seen for P. aeruginosa and L. monocytogenes, resulting in a log reduction in excess of 4 logs for P. aeruginosa and 5 logs for L. monocytogenes at 40 g/m3. This is in excess of the 4 log reduction required in the European Surface Test (EN 13697) for surface disinfectants. S. aureus was significantly more resistant than the other vegetative organisms P. aeruginosa and L. monocytogenes, and its log reduction was not increased at 30 or 40 g/m3.
With ozone, as with HPV, there is a relationship between concentration and log reduction, with differences in resistance being expressed by strain types. At 20ppm ozone, S. aureus was most resistant followed by P. aeruginosa, and with L. monocytogenes being most sensitive. A reduction of >4 logs was achieved with L. monocytogenes at an ozone concentration of 20 ppm, which equates to the requirements of the European Surface Test (EN 13697) for chemical disinfectants. A reduction approaching 4 logs was also achieved with P. aeruginosa. The reason for the resistance of S. aureus to HPV and ozone is unclear, but may be due to the tendency of S. aureus cells to clump together, which may resist chemical vapours.
As an overall conclusion from the laboratory trials, HPV and ozone have several advantages as they can effectively penetrate every part of a room, including sites that might prove difficult to gain access to with conventional liquids, applied either by fogging or via manual disinfection procedures. High concentrations of HPV and ozone may be able to control pathogens such as Listeria in the food processing environments if the high log reductions obtained in the laboratory (e.g. >5 log reductions) can be obtained in the field. The major disadvantage of using HPV and ozone is the potential toxicity at high concentrations, which precludes using them in areas where people are working. The technique can therefore only be used in areas that can be isolated and sealed off during the decontamination process and when appropriate health and safety risk assessments are undertaken and adhered to.
Nevertheless, it is clear that with appropriate controls, periodic application using these techniques has the possibility of eliminating pathogens from the food processing environment and/or limiting their persistence. Further trials are needed, however, to support this in practice.
References
1. Holah, J., Bird, J & HallL, K. (2004) The microbial ecology of high risk, chilled food factories; evidence for persistent Listeria spp. And Escherichia coli strains. Journal of Applied Microbiology 97, 68-77
2. Holah, J., Bird, J. & Hall, K. (2004) Listeria monocytogenes and Escherichia coli in high risk, chilled food factories; where do they come from? R&D Report No.199, Campden BRI
3. EN 13697:2001 Chemical disinfectants and antiseptics – Quantitative non-porous surface test for the evaluation of bactericidal and/or fungicidal activity of chemical disinfectants used in food, industrial, domestic and institutional areas – Test methods and requirements without mechanical action (phase 2/step 2) http://www.bsigroup.com/
4. Burfoot, D., Hall, K., Brown, K. & Xu, Y. (1999) Fogging for the disinfection of food processing factories and equipment. Trends in Food Science & Technology, 10 (6-7), 205-210
About the Authors
Alicja Malinowska
Alicja Malinowska is a Research Officer in the Food Hygiene Department at Campden BRI with an engineering degree in Food Quality and Management and a Masters degree in Food Technology and Nutrition from the Wrocław University of Environmental and Life Science in Poland. Alicja based her Master thesis on Campden BRI’s three year project on ‘The influence of electrostatic charge on microbial contamination of PET bottles’ and has contributed Campden BRI R&D reports on clean filling and whole room disinfection. Alicja has worked at Campden BRI for over five years and is currently responsible for managing the day to day activities of a number of research and contract projects. This has involved laboratory based research work, factory studies and presentations at internal Campden BRI and international meetings and conferences. Current research projects include ‘Sustainable water use in the food chain’ and ‘Effective packaging surface decontamination’.
John Holah
Dr. John Holah is an applied micro – biologist and Head of the Food Hygiene Department at Campden BRI working both in food factories and in the laboratory. He has a wide knowledge of the food industry and conducts troubleshooting audits for food factories and catering establishments all over the world investigating microbial and foreign body contamination incidents and problem solving. His Department has expertise on the hygienic design of food factories, production layout, and food processing equipment; aerobiology and factory air handling systems; factory services and water systems; cleaning and disinfection; personnel Figure 6 Effect of gaseous O hygiene and environmental sampling.