The role of microstructure in texture perception
- Like
- Digg
- Del
- Tumblr
- VKontakte
- Buffer
- Love This
- Odnoklassniki
- Meneame
- Blogger
- Amazon
- Yahoo Mail
- Gmail
- AOL
- Newsvine
- HackerNews
- Evernote
- MySpace
- Mail.ru
- Viadeo
- Line
- Comments
- Yummly
- SMS
- Viber
- Telegram
- Subscribe
- Skype
- Facebook Messenger
- Kakao
- LiveJournal
- Yammer
- Edgar
- Fintel
- Mix
- Instapaper
- Copy Link
Posted: 13 May 2011 | Fred van de Velde & H. Jan Klok, NIZO Protein Centre, NIZO food research and Tristan Laundon & E. Allen Foegeding, North Carolina State University, Department of Food, Bioprocessing and Nutrition Sciences | No comments yet
Foods are eaten not only for their nutritional value but also for the pleasure of eating. Food producers reformulate their products to comply with consumer trends on fat, salt and sugar reduction as well as to reduce the number of additives. The number one goal is to maintain consumer acceptance. Understanding the role and interactions of ingredients in the texture and stability of foods is of key importance to develop improved food products. This article describes the role of microstructure in understanding the ingredient functionality in food products.
Composite food products are complex products composed of a wide range of ingredients. The three main ingredients (also called macronutrients) are proteins, carbohydrates and fats/oils. The type or origin of the ingredients, their concentrations and the applied processing determines the final product properties and sensory profile. For example, milk can be transformed into different products such as yoghurt, cheese, butter and desserts just by changing processing and ingredients.
Foods are eaten not only for their nutritional value but also for the pleasure of eating. Food producers reformulate their products to comply with consumer trends on fat, salt and sugar reduction as well as to reduce the number of additives. The number one goal is to maintain consumer acceptance. Understanding the role and interactions of ingredients in the texture and stability of foods is of key importance to develop improved food products. This article describes the role of microstructure in understanding the ingredient functionality in food products. Composite food products are complex products composed of a wide range of ingredients. The three main ingredients (also called macronutrients) are proteins, carbohydrates and fats/oils. The type or origin of the ingredients, their concentrations and the applied processing determines the final product properties and sensory profile. For example, milk can be transformed into different products such as yoghurt, cheese, butter and desserts just by changing processing and ingredients.
Foods are eaten not only for their nutritional value but also for the pleasure of eating. Food producers reformulate their products to comply with consumer trends on fat, salt and sugar reduction as well as to reduce the number of additives. The number one goal is to maintain consumer acceptance. Understanding the role and interactions of ingredients in the texture and stability of foods is of key importance to develop improved food products. This article describes the role of microstructure in understanding the ingredient functionality in food products.
Composite food products are complex products composed of a wide range of ingredients. The three main ingredients (also called macronutrients) are proteins, carbohydrates and fats/oils. The type or origin of the ingredients, their concentrations and the applied processing determines the final product properties and sensory profile. For example, milk can be transformed into different products such as yoghurt, cheese, butter and desserts just by changing processing and ingredients. And wheat flour can be turned into bread, cake, pasta and many more products. Current consumer trends force the food manufacturers to reformulate their products to reduce the fat, salt and sugar content or to reduce the number of additives. New product development is often a cyclic process of changes in recipe, ingredients and processing followed by instrumental and sensory analysis to judge the changes. Understanding the role and interaction of the different ingredients in the food product can reduce the development time significantly. The microstructure of the food product is an important aspect in understanding the role of ingredients on the texture, stability and sensory perception of food products.
Confocal Laser Scanning Microscopy (CLSM) is a powerful tool to visualise the microstructure (i.e., structures seen on the scale of micrometers) of food products as well as the special distribution of ingredients therein. CLSM imaging is a microscopic technique that adds two main advantages to regular light microscopy. Firstly, the confocal principle makes an optical section from the product under study avoiding the laborious slicing of samples. Secondly, the use of laser light in combination with fluorescent dyes identifies different ingredients in the microstructure of a product. Thus, different ingredients (e.g. fat, protein, starch) can simultaneously be visualised within the microstructure of an undisturbed food product. The textbox ‘Different foods, different microstructures’ shows some characteristic microstructures of foods across different product categories and the role of the ingredients therein.
Learning from model systems
Real food products can be visualised by CLSM and the relationship between microstructure, texture and perception can be studied using products with different compositions and properties. However, model systems allow a higher degree of freedom and flexibility. Protein gels form the starting point for the development of model systems. The textural properties of protein gels can be modulated by the concentration of proteins, solvent quality, such as pH and ionic strength and gelation method. Extra dimensions are created by the addition of emulsion droplets or by mixing polysaccharides. Mixed protein polysaccharide gels show microphase separation on the micrometer level (observed by CLSM) resulting in gels with different microstructures and mechanical properties1,2. Clear relationships between microstructure, texture and perception have been described for mouthfeel attributes, such as hardness (or firmness), crumbliness, separation (or juiciness) and spreadable3,4.
The attribute crumbliness is of special interest as this attribute is associated with the undesired texture of (very) low-fat cheeses5. The crumbly perception of the mixed protein/polysaccharide gels is defined by the sensory panel as follows: “The sample falls apart in pieces due to compression between tongue and palate.” It turned out that the crumbly score is positively correlated with the amount of energy that can be stored in the material during deformation. In other words, the more energy is dissipated during deformation (compression between tongue and palate or during mastication), the lower the crumbly score. A fully elastic material will store most of the energy and will at fracture spontaneously fall apart in pieces. A high viscous material will dissipate most of the energy and will disintegrate into a more homogeneous mass. Most foods products are visco-elastic materials and show intermediate energy storage. The amount of energy that can be stored in a food product is instrumentally measured by the Recoverable Energy measurement (RE). In this measurement, the material is compressed (but not fractured) and subsequently decompressed. The energy release during the decompression step is related to the energy needed to compress the sample. The ratio between these two energy terms is the recoverable energy.
Energy can be dissipated by friction, internal viscous flow or flow of fluids out of the gel. In mixed protein/polysaccharide gels, the recoverable energy correlates well with the crumbly score given by a trained sensory panel (Figure 1). Moreover, the recoverable energy related to the amount of serum release during compression, which in turn is a result of the microstructure of the model system. In conclusion, the flow of serum through the matrix causes energy dissipation during compression of the product, which resulted in low energy storage and thereby a low crumbly score6. In the case of emulsion-filled gels, the friction between the emulsion droplets and the gel matrix is the origin of the energy dissipation7. The interaction between the emulsion droplets and the matrix is of crucial importance to emulsion filled gels. Emulsion droplets can be bound to the matrix by covalent or electrostatic interactions and, thereby, behave as so-called active fillers. The filler particle becomes a structural element of the gel matrix and contributes to gel firmness. The opposite are unbound droplets that do not interact with the matrix (for example, emulsion droplets coated with a non-charged emulsifier, Tween, in a gelled protein matrix). As unbound droplets can ‘freely’ move in the void of the gel, during deformation of the matrix, they will come in contact with the matrix and dissipate energy by friction. Bound droplets do not have this flexibility to move and will generate less friction. As a result, the decrease in recoverable energy by increasing oil volume effect will be more pronounced for unbound droplets compared to bound droplets (Figure 3). Thus, by reducing the fat content in emulsion-filled gels, the energy storage and dissipation changes and thereby the crumbly perception will increase.
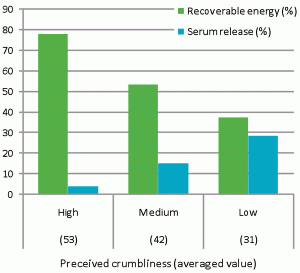
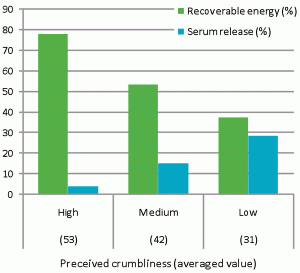
Figure 1 Relationship between crumbliness perception and instrumentally measured recoverable energy and serum release for three groups of mixed protein / polysaccharide gels with distinct behaviour
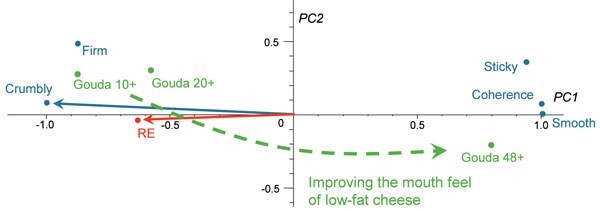
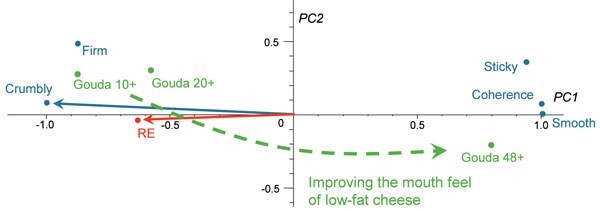
Figure 2 Sensory space (PCA diagram) of Gouda cheese variants with different fat levels (Blue: sensory attributes; Red: Instrumental measurement; Green: samples)
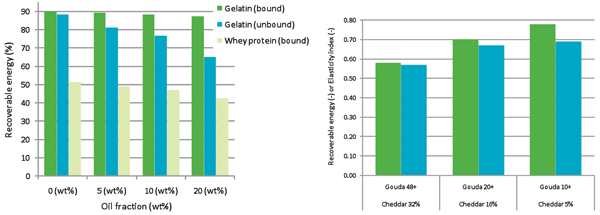
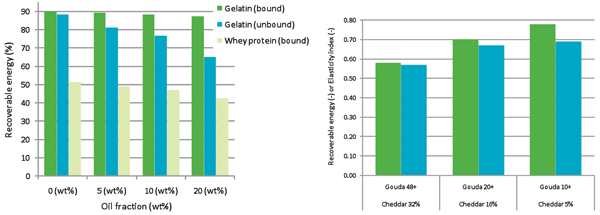
Figure 3 Recoverable energy of gelatine and whey protein gels with bound and unbound droplets (left) and recoverable energy and elasticity index of Gouda cheese and Cheddar cheese with different fat levels (right)
The role of fat in cheese
Cheese is considered as an emulsion-filled gel. The matrix is formed by the fused casein micelles and the fat globules are the fillers. In semi-hard cheeses, such as Gouda and Cheddar, the casein matrix is clearly a visco-elastic material, which properties are determined by the presence of the emulsion droplets (fat globules). As shown, the interaction between the emulsion droplets and matrix is of importance to understand the impact of the droplets on the texture of the product. In cheese prepared from non-homogenised milk, the fat globules are stabilised with the native milk fat globule membrane. The native milk fat globule membrane is rich in phospholipids and can be considered as unbound fillers that weaken the network structure. Milk fat globules acts as structure breakers in the casein matrix of cheese. Upon fat reduction, the texture of cheese is perceived as more firm, rubbery and crumbly (Figure 2 opposite). In addition, homo – genisation of the cheese milk results in an increased firmness and rubberiness of the cheese, as the native milk fat globule membrane is replaced by milk proteins upon homogenisation. As a consequence, the fat globules (emulsion droplets) turn into bound ‘active’ fillers that reinforce the casein matrix resulting in a firm and rubbery texture.
Returning to the fat reduction in cheese, Figure 3 opposite shows that fat reduction in Gouda cheese increases the crumbly scores. As part of a study on the impact of fat reduction in Gouda cheese on the mechanical properties, the recoverable energy of these cheese variants was measured5. It turned out that recoverable energy increased by decreasing the fat content (Figure 3). A comparable trend was observed for the elasticity index of Cheddar cheeses with different fat levels (Figure 3). The elasticity index is an indicator for the elastically stored energy measured by large strain torsion analyses8. Concluding, independent of the cheese type (Gouda and Cheddar) and the analytical methods (compression or torsion), the elastically stored energy increased with decreasing fat content. The fat globules in semi-hard cheese act as structure breakers in the casein network allowing for energy dissipation leading to a smooth and coherent cheese texture. In addition to the role as structure breaker, the coalescence of the fat globules plays an important role in the perception of full-fat cheese. The coalescence of the fat globules was observed during the rheo-microscopic investigation of low- and full-fat Cheddar samples (Textbox “Micro – structures under deformation).
Conclusions
Confocal Laser Scanning Microscopy (CLSM) is a powerful tool to visualise the microstructure (i.e., structures seen on the scale of micrometers) of food products. This technology has allowed understanding relationships between ingredients, processing, texture and perception of semi- and soft-solid foods. Relationships observed in model systems, such as mixed protein/polysaccharide gels or emulsion-filled gels, also apply for real foods. Understanding the role of fat as structure breaker in cheese allows designing new ingredients or processing routes to develop low-fat cheeses with an excellent texture and mouthfeel. This shift in texture is indicated with the dashed arrow in Figure 2 (page 54).
References
1. de Jong, S. and F. van de Velde (2007) Charge density of polysaccharides controls microstructure and large deformation properties of mixed gels. Food Hydrocolloids 21, 1172-1187
2. Çakir, E. and E.A. Foegeding (2011) Combining protein micro-phase separation and proteinpolysaccharide segregative phase separation to produce gel structures. Food Hydrocolloids (2011), doi: 10.1016/j.foodhyd.2011.02.022
3. van den Berg, L., T. van Vliet, E. van der Linden, M.A.J.S. van Boekel and F. van de Velde (2008a) Physical properties giving the sensory perception of whey proteins/polysaccharide gels. Food Biophysics 3, 198-206
4. Foegeding, E.A., Daubert, C.R., Drake, M.A., Essick, G., Trulsson, M., Vinyard, C. and F. van de Velde (2011) A comprehensive approach to understanding textural properties of semi- and soft-solid foods. Journal of Texture Studies (2011), doi: 10.1111/j.1745- 4603.2011.00286x
5. Düsterhöft, E.-M. and F. van de Velde (2008) Texture properties of cheese related to oral and industrial processing. Oral presentation during the 5th IDF Symposium on Cheese Ripening, Bern, March 2008
6. van den Berg, L., A.L. Carolas, T. van Vliet, E. van der Linden, M.A.J.S. van Boekel and F. van de Velde (2008b) Energy storage controls crumbly perception in whey proteins/polysaccharide mixed gels. Food Hydrocolloids 22, 1404-1417
7. Sala, G., T. van Vliet, M. Cohen Stuart, G.A. van Aken, F. van de Velde (2009) Deformation and fracture of emulsion-filled gels: Effect of oil content and deformation speed. Food Hydrocolloids 23, 1381-1393
8. Rogers, N.R., Drake, M.A., Daubert, C.R., McMahon, D.J., Bletsch, T.K., and E. A. Foegeding (2009) The effect of aging on low-fat, reduced-fat, and full-fat Cheddar cheese texture. Journal of Dairy Science 92, 4756-4772
9. van den Berg, L., H.J. Klok, T. van Vliet, E. van der Linden, M.A.J.S. van Boekel and F. van de Velde (2008c) Quantification of a 3D structural evolution of food composites under large deformations using microrheology. Food Hydrocolloids 22, 1574-1583
10. Berry, T.K., H.J. Klok, D.J. McMahon, F. van de Velde and E.A. Foegeding (2009) Structure of low and full-fat Cheddar cheese as determined by microrheology. Poster presentation during the 6th NIZO Dairy Conference – Dairy Ingredients: Innovations in functionality. Papendal, the Netherlands, September 30 – October 2, 2009
About the Author
Fred van de Velde is project manager and group leader Ingredient Technology at NIZO food research. NIZO food research is an independent research company. Fred studied organic chemistry at the Delft University of Technology. He joined NIZO food research in 2002 after a post-doc position within the Wageningen Centre for Food Sciences. He is expert on food microstructure and its relationship with mechanical properties and sensory perception.
H. Jan Klok is a research specialist at NIZO food research. He started at NIZO 35 years ago after finishing laboratory education. Jan Klok is expert on microscopy, light scattering, differential scanning calorymetry. He is active in projects on fat and protein functionality and powder technology.
Tristan Laundon received both her Bachelor of Science and Master of Science degrees in Food Science from North Carolina State University. After completing her MSc degree, Tristan remained at the university, doing research for Dr. E. Allen Foegeding, including work imaging cheddar cheese structure and investigating cheese microrheology. She currently works for the university’s Entrepreneurial Program, which involves food product testing and nutritional labelling for customers working to start their own food businesses.
Dr. E. Allen Foegeding is a William Neal Reynolds Distinguished Professor in the Department of Food, Bioprocessing and Nutrition Sciences at North Carolina State University. He conducts research and teaches in the areas of protein functionality and polymer and colloidal properties of food products, with emphasis on whey proteins and dairy products. Dr. Foegeding is a fellow of the Agricultural and Food Chemistry Division of the American Chemical Society and the Institute of Food Technologists. He is the recipient of numerous research and teaching awards, including the 2003 Research and Development Award and the 2010 William V. Cruess teaching award from the Institute of Food Technologists. He has supervised 50 graduate students and published over 150 refereed articles.