Emerging technologies for food refrigeration applications
- Like
- Digg
- Del
- Tumblr
- VKontakte
- Buffer
- Love This
- Odnoklassniki
- Meneame
- Blogger
- Amazon
- Yahoo Mail
- Gmail
- AOL
- Newsvine
- HackerNews
- Evernote
- MySpace
- Mail.ru
- Viadeo
- Line
- Comments
- Yummly
- SMS
- Viber
- Telegram
- Subscribe
- Skype
- Facebook Messenger
- Kakao
- LiveJournal
- Yammer
- Edgar
- Fintel
- Mix
- Instapaper
- Copy Link
Posted: 13 May 2011 | Savvas Tassou, Head of School of Engineering and Design, Brunel University | No comments yet
Refrigeration is used in all stages of the food chain, from food processing to distribution, retail and final consumption in the home. The food industry employs both chilling and freezing processes where the food is cooled from ambient to temperatures above 0°C in the former and between -18°C and -35°C in the latter to slow the physical, microbiological and chemical activities that cause deterioration in foods. In these processes, mechanical refrigeration technologies are invariably employed that contribute significantly to the environmental impacts of the food sector both through direct and indirect greenhouse gas emissions. To reduce these emissions, research and development worldwide is aimed at both improving the performance of conventional systems and the development of new refrigeration technologies of potentially much lower environmental impacts.
Refrigeration is used in all stages of the food chain, from food processing to distribution, retail and final consumption in the home. The food industry employs both chilling and freezing processes where the food is cooled from ambient to temperatures above 0°C in the former and between -18°C and -35°C in the latter to slow the physical, microbiological and chemical activities that cause deterioration in foods. In these processes, mechanical refrigeration technologies are invariably employed that contribute significantly to the environmental impacts of the food sector both through direct and indirect greenhouse gas emissions. To reduce these emissions, research and development worldwide is aimed at both improving the performance of conventional systems and the development of new refrigeration technologies of potentially much lower environmental impacts.
Refrigeration is used in all stages of the food chain, from food processing to distribution, retail and final consumption in the home. The food industry employs both chilling and freezing processes where the food is cooled from ambient to temperatures above 0°C in the former and between -18°C and -35°C in the latter to slow the physical, microbiological and chemical activities that cause deterioration in foods. In these processes, mechanical refrigeration technologies are invariably employed that contribute significantly to the environmental impacts of the food sector both through direct and indirect greenhouse gas emissions. To reduce these emissions, research and development worldwide is aimed at both improving the performance of conventional systems and the development of new refrigeration technologies of potentially much lower environmental impacts.
Since the emergence of chlorofluorocarbon (CFC) and hydrofluorocarbon (HCFC) refrigerants in the 1930’s, the vapour compression refrigeration cycle has gained dominance over alternative cooling technologies. In the 1980’s, however, increased environmental awareness and the realisation of the impact of CFC emissions on the ozone layer has prompted international agreements that led to the ban of CFCs and the establishment of time-scales for the phase-out of HCFCs. Even though new refrigerants, namely HFCs, have been developed with zero Ozone Depletion Potential (ODP), these refrigerants invariably have high Global Warming Potential (GWP) and make significant contributions to greenhouse gas emissions both directly through refrigerant leakage and indirectly through emissions from power stations that generate the electrical energy required to drive them. This has renewed interest in thermally driven technologies and the development of new and innovative technologies that could offer both economic and environmental advantages over the conventional vapour compression cycle in the future. This article provides a brief review of these technologies and their potential for application in the food sector.
Sorption refrigeration – adsorption systems
Sorption refrigeration technologies such as absorption and/or adsorption are thermally driven systems, in which the conventional mechanical compressor of the common vapour compression cycle is replaced by a ‘thermal compressor’ and a sorbent. The sorbent can be either solid in the case of adsorption systems or liquid for absorption systems. When the sorbent is heated, it desorbs the refrigerant vapour at the condenser pressure. The vapour is then liquefied in the condenser, flows through an expansion valve and enters the evaporator. When the sorbent is cooled, it reabsorbs vapour and thus maintains low pressure in the evaporator. The liquefied refrigerant in the evaporator absorbs heat from the refrigerated space and vaporises, producing the cooling effect.
Adsorption refrigeration, unlike absorption and vapour compression systems, is an inherently cyclical process and multiple adsorbent beds are necessary to provide approximately continuous capacity. Adsorption systems are already commercially available for air conditioning applications from a small number of manufacturers with capacities between 70kW and 1300kW capable of being driven by low grade heat 50 – 90°C and able to give COPs of about 0.71. Research and development is also underway to produce systems for refrigeration applications. Research prototypes for refrigeration temperatures down to -25°C are currently in operation or under development2. Applications in the food sector will primarily be in areas where waste heat is available to drive the adsorption system. Such applications can be found in food factories and transport refrigeration. Another possible application is in tri-generation where adsorption systems can be used in conjunction with combined heat and power systems to provide refrigeration.
Ejector refrigeration systems
Ejector or jet pump refrigeration is a thermally driven technology that has been used for cooling applications for many years. Their greatest advantage is their capability to produce refrigeration using waste heat or solar energy as a heat source at temperatures above 80°C. Systems have been developed with cooling capacities ranging from a few kilowatts to 60 MW but despite extensive development efforts, the COP of the system, which can be defined as the ratio of the refrigeration effect to the heat input to the boiler, is still relatively low at less than 0.2. Applications in the food sector will primarily be in areas where waste heat is available to drive the ejector system. Such applications can be found in food processing factories where the ejector refrigeration system can be used for product and process cooling and transport refrigeration.
Air cycle refrigeration
Air cycle systems can produce low temperatures for refrigeration by subjecting the gaseous refrigerant (air) to a sequence of processes comprising compression, followed by constant pressure cooling, and then expansion to the original pressure to achieve a final temperature lower than at the start of compression. Air cycle refrigeration is based on the reversed Joule (or Brayton) cycle illustrated in Figure 1.
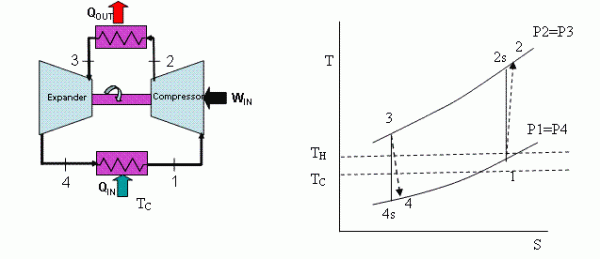
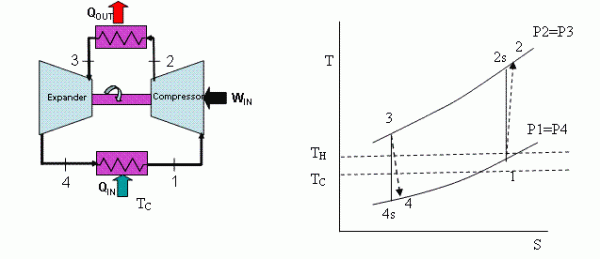
Figure 1 Reversed Joule Cycle
Air cycle is a reasonably well established technology. Plant operating characteristics are understood and issues such as condensation and icing have been addressed and solutions developed. Closed and open air cycle systems have been developed by industrial companies with refrigeration capacities ranging from 11 to 700 kW for closed systems and from 15 to 300kW for open systems3. Information on the coefficient of performance for refrigeration is sparse but most values quoted are in the range between 0.4 to 0.7. It is also noted that the efficiency of air cycle systems is relatively unaffected under part load conditions.
Air cycle refrigeration can deliver air temperatures down to -100°C, giving it a niche position in the – 50°C to – 100°C range, beyond the capability of a vapour compression plant. Air cycles also generate high air temperatures, typically of over 200°C, that can be used in combination with the low temperatures to integrate cooking and refrigeration processes.
In the food sector air cycle technology can be applied to rapid chilling and/or freezing (including air blast, tunnel, spiral, fluidised bed and rotary tumble equipment), for refrigerated transport and for integrated rapid heating and cooling4,5.
Trigeneration
Tri-generation technology is a technology that can simultaneously provide three forms of output energy; electrical power, heating and cooling6. In essence, trigeneration systems are CHP (Combined Heat and Power) or co-generation systems, integrated with a thermally driven refrigeration system to provide cooling as well as electrical power and heating (Figure 2).
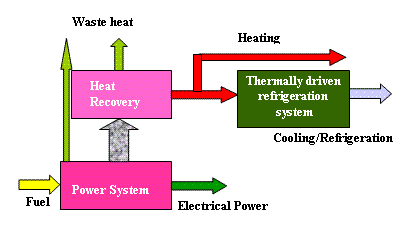
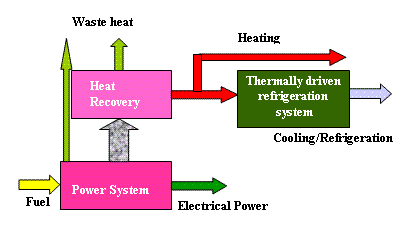
Figure 2 Schematic of a trigeneration system
Trigeneration systems can have overall efficiencies as high as 90 per cent compared to 33 -35 per cent for electricity generated in central power plants. Trigeneration systems have been in operation for many years7. Developments in recent years have mainly concentrated on individual subsystems such as the power system, heat recovery system, thermally driven refrigeration machines and system integration and control.
There are a number of examples of application of trigeneration plants in the food manufacturing industry. More recently, application of trigeneration has been extended to supermarkets with a very small number of installations in the USA, the UK and Japan.
Stirling cycle refrigeration
The Stirling cycle cooler is a closed-cycle regenerative thermal machine in which gas is shuttled backwards and forwards between the hot end and cold end spaces of the system by a piston and a displacer (Figure 3), so that the temperature of the system during compression is, on average, higher than during expansion. The heat generated in the cycle is rejected through a heat exchanger at the hot end and heat is absorbed from the space to be cooled via a heat exchanger at the cold end. The Stirling cycle was first commercially employed for refrigeration in the 1950s by the Philips company in Eindhoven. Little development was done on the Stirling cycle for higher temperature commercial refrigeration applications until the 1990s, which saw the beginning of the development of free-piston Stirling coolers.
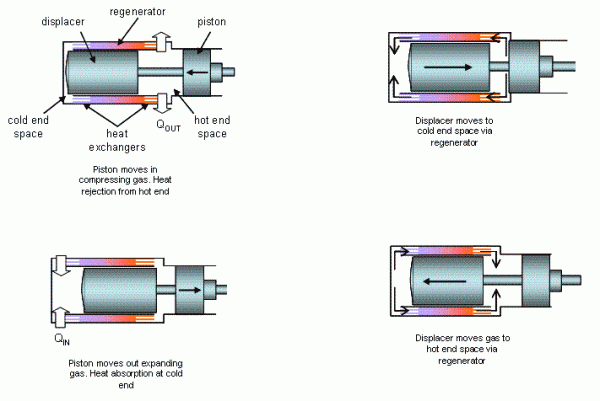
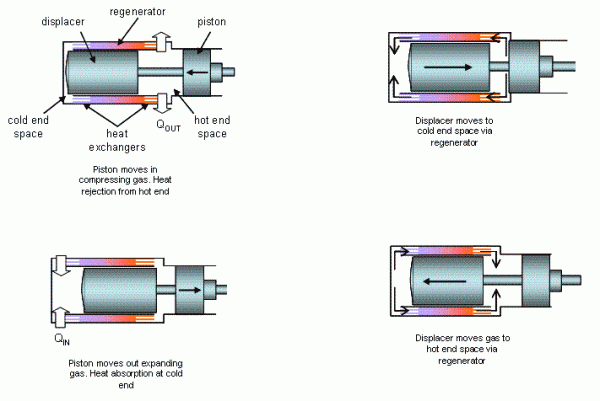
Figure 3 Piston and Displacer movements during Stirling refrigeration cycle
FPSC units with nominal maximum cooling capacities of 40W and 100W have been produced, with larger capacity units, up to 300W, reported to be under development8. FPSC based products, including freezer boxes and a system for the marine refrigeration market, have been developed by licensees. Coefficients of performance measured for FPSCs with warm head temperatures close to 30°C vary with the cold head temperature. Values of COP between two and three have been reported for cold head temperatures around 0°C, and values around one for cold head temperatures approaching – 40°C. FPSCs can operate down to cryogenic temperatures and hence can be used in many food refrigeration applications. The market for FPSCs in the food sector is likely to be domestic and portable refrigerators and freezers as well as beverage can vending machines and other integral refrigerated display equipment.
Thermoelectric refrigeration
Thermoelectric cooling devices utilise the Peltier effect, whereby the passage of a direct electric current through the junction of two dissimilar conducting materials causes the junction to either cool down (absorbing heat) or warm up (rejecting heat), depending on the direction of the current (Figure 4).
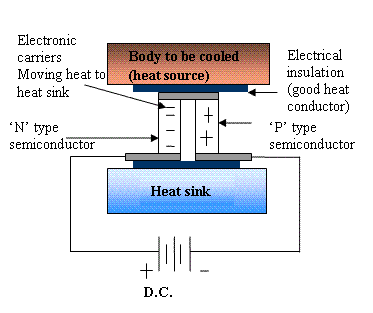
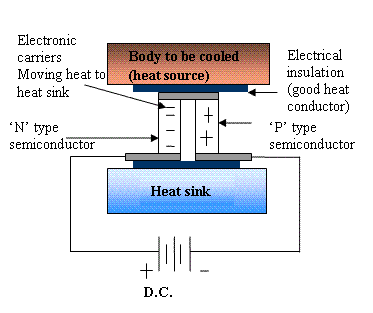
Figure 4 Thermoelectric cooling (or Peltier) couple
Thermoelectric modules are available commercially to suit a wide range of small and medium cooling duties. In a thermoelectric refrigeration system, the Peltier module (or modules) must be interfaced with heat exchange systems to facilitate heat removal from the refrigerated space to the cold-side and heat rejection from the hot-side to the surroundings. The thermal resistances introduced by the heat exchange systems have a significant influence on the overall COP of the system.
Thermoelectric cooling systems offer advantages of no moving parts and good reliability, absence of noise and vibration, compactness and low weight. However, they have lower COP and higher capital cost than vapour compression systems9. Current applications in the food sector include hotel room (mini-bar) refrigerators, refrigerators for mobile homes, trucks, recreational vehicles and cars, portable picnic coolers, wine coolers, beverage can coolers and drinking water coolers. Other potential future applications include domestic and commercial refrigerators and freezers, and mobile refrigeration and cooling systems.
Thermoacoustic refrigeration
Thermoacoustic refrigeration systems operate by using sound waves and a non-flammable mixture of inert gas (helium, argon, air) in a resonator to produce cooling. Thermoacoustic devices are typically characterised as either ‘standing-wave’ or ‘travelling-wave’10. A schematic diagram of a standing wave device is shown in Figure 5.
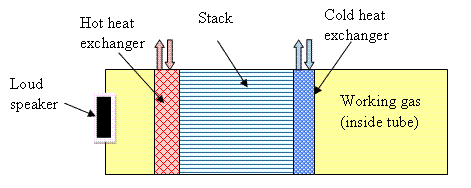
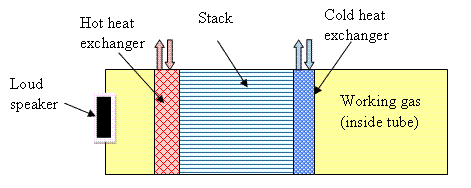
Figure 5 Sound wave thermoacoustic refrigerator
The main components are a closed cylinder, an acoustic driver, a porous component called a ‘stack’, and two heat-exchanger systems. Application of acoustic waves through a driver such as a loud speaker makes the gas resonant. As the gas oscillates back and forth, it creates a temperature difference along the length of the stack. The temperature difference is used to remove heat from the cold side and reject it at the hot side of the system. In the travelling-wave device, the pressure is created with a moving piston and the conversion of acoustic power to heat occurs in a regenerator rather than a stack.
Thermoacoustic refrigerators have the potential to cover the whole spectrum of refrigeration down to cryogenic temperatures. A number of design concepts and prototypes are under development in many research establishments. Research effort is currently directed to the development of flow-through designs (open systems) which will reduce or eliminated the use of heat exchangers. It is likely that potential market for food applications will initially be in the low capacity equipment such as domestic and commercial refrigerators, freezers and cabinets.
Magnetic refrigeration
A magnetic refrigeration cycle employs a solidstate magnetic material as the working refrigerant, and exploits the magnetocaloric effect (MCE), the ability of a material to warm-up in the presence of a magnetic field and cool down when the field is removed. Magnetisation and demagnetisation of a magnetic refrigerant can be viewed as analogous to compression and expansion in a vapour compression refrigeration cycle, but in contrast, these magnetic processes are virtually loss-free and reversible. Further advantages associated with the solid-state nature of magnetic refrigerants are zero ODP and zero GWP which offer the prospect of efficient, environmentally friendly and compact cooling11.
Magnetic refrigeration technology is under active development and a number of prototype systems (including both reciprocating and rotary designs) have been announced. Cooling capacities of prototypes are low, with a COP of 1.8 at room temperature12.
Magnetic refrigeration has the potential for use across the whole refrigeration temperature range, down to cryogenic temperatures but further research and development is still required for the development of materials with high magnetocaloric effect, to reduce the size, weight and cost of the system. It is anticipated that the first commercial applications will be for low capacity stationary and mobile refrigeration systems.
Summary and conclusions
This section summarises approaches and future technologies that could be used to reduce the energy consumption and GHG emissions of the refrigeration of food.
Transport refrigeration: In transport refrigeration, there are opportunities to reduce thermal loads through better insulation materials such as vacuum insulation, and the size and energy use of the refrigeration system on the truck through thermal energy storage based on phase change materials (PCMs) that can be charged at base. Ice slurries are also under consideration for thermal storage in chilled distribution. Total loss systems (cryocoolers) have also been re-evaluated as a replacement of vapour compression systems. Other possible systems include air cycle, hybrid and solar powered systems and recovery of thermal energy from the engine exhaust and use it to drive sorption systems, ejector systems, thermoacoustic refrigerators and or/for power generation using thermoelectrics or turbogenerators.
Integral refrigeration equipment (cabinets): Hydrocarbons are already being used as a replacement refrigerant for HFCs in many integral refrigerated cabinets. CO2 systems have also been developed and a small number of integral CO2 cabinets are now in service. Stirling cycle coolers are already commercially available and reduction in cost accompanied by efficiency improvements can make them serious contenders for cabinet refrigeration systems. Other candidate technologies approaching commercialisation are thermoelectric, thermoacoustic and magnetic refrigeration.
Supermarket refrigeration systems: The environmental impacts of supermarket refrigeration systems can be reduced through the improvement of equipment efficiencies, reduction in the refrigerant charge and reduction or elimination of refrigerant leakage. There are also opportunities for thermal integration of refrigeration and HVAC systems and the application of CHP and trigeneration technologies. CO2 based systems are also fast gaining in popularity and a number of different system configurations have already been adopted.
Food processing: Ammonia vapour compression systems are dominant in food processing. Plant energy savings can be achieved through improvements in component design and control and heat recovery. Possible system alternatives include CO2 systems and CO2 / R717 cascade systems. Air cycle technology offers potential for low temperatures, below 50°C and for combined heating and cooling. Other possible approaches include the recovery and use of waste heat for refrigeration through sorption and ejector systems and for power generation (thermoelectric, Stirling, thermoacoustic, turbogenerators). There may also be possibilities for the use of biomass which may be a bi-product of food processing for CHP and trigeneration.
Food storage (cold stores): Large food storage facilities normally employ ammonia vapour compression plant and this is likely to continue in the future. Another possibility that offers heat recovery potential is the use of CO2 as a refrigerant on its own or in combination with ammonia in a CO2 /R717 cascade arrangement. Because of their location, normally in not densely populated areas, food storage facilities offer potential for the use of biomass for combined heat and power or trigeneration. A small number of such plants are already in operation. Large food storage facilities also offer potential for the use of wind power and solar energy to generate electricity to drive vapour compression equipment and/or heat for sorption systems.
Acknowledgements
Work presented in this paper was carried out with financial support from the Department of Environment, Food and Rural Affairs (Defra).
References
1. S.A.Tassou, J.S.Lewis, Y.T. Ge, A. Hadawey, I. Chaer. A review of emrging technologis for food refrigeration applications, Applied Thermal Engineering 30 (2010) 263–276
2. Z. S. Lu, R.Z. Wang, L.W. Wang, C.J. Chen. Performance analysis of an adsorption refrigerator using, Carbon 44 (2006) 747–752
3. S. Kikuchi, S. Okuda, H. Igawa, S. Morii, M. Mitsuhashi and H. Higashimori, Development of air cycle system for refrigeration, Mitsubishi Heavy Industries Technical Review, Vol. 42, No. 4, 1-4, November 2005
4. S. W. T. Spence, W. J. Doran, D. W. Artt, G. McCullough, Performance analysis of a feasible air-cycle refrigeration system for road transport, International Journal of Refrigeration, 27, (2005), 381-388
5. J. Evans, A. Gigiel, T. Brown, Development of integrated, rapid heating and cooling systems for the food industry using air cycle technologies, 7th IIR Gustav Lorentzen Conference on Natural Working Fluids, Trondheim, May 28-31, 2006
6. S. A. Tassou, I. Chaer, N. Sugiartha, Y. T Ge, D. Marriott (2007) Application of tri-generation systems to the food retail industry, Energy Conversion and Management, Vol.48, 11, 2988-2995
7. Bassols, J., Kuckelkorn, B., Langreck, J., Schneider, R., Veelken, H., Trigeneration in the food industry, Applied Thermal Engineering, 2002, Volume 22, Issue 6, Pages 595-602
8. N.W. Lane, Commercialization status of free-piston Stirling machines, 12th International Stirling Engine Conference, Durham, UK, September 2005
9. P.K. Bansal and A. Martin, Comparative study of vapour compression, thermoelectric and absorption refrigerators, International Journal of Energy Research, 24, 93-107, 2000
10. T. C. Bammann, C. Q. Howard, B. S. Cazzolato, Review of flow-through design in thermoacoustic refrigeration, Proceedings of ACOUSTICS 2005, 9-11 November 2005, Busselton, Western Australia
11. V.K. Pecharsky and K.A. Gschneidner, Jr, Advanced magnetocaloric materials: What does the future hold? International Journal of Refrigeration, 29, 1239-1249, 2006
12. Chubu Electric Power Co. Inc., Development of room temperature magnetic refrigeration system – world leading performance a big step forward in achieving practical systems, Press Release, 7 November 2006 (available at http://www.chuden.co.jp/english/ corporate/press2006/1107_1.html
About the Author
Professor Savvas Tassou is the Head of the School of Engineering and Design at Brunel University. Professor Tassou has over 30 years research experience in energy systems and in particular, refrigeration and heat pumping technologies. Over the last 10 years, the work has focused on energy conservation in the food chain and in particular the reduction of the environmental impacts of food refrigeration equipment. Current externally funded research includes the design and optimisation of refrigeration systems employing natural refrigerants such as hydrocarbons and CO2 and the integration and application of trigenertion and CO2 refrigeration systems in the food industry.