Emerging challenges and trends in UHT processing of milk and other dairy products
- Like
- Digg
- Del
- Tumblr
- VKontakte
- Buffer
- Love This
- Odnoklassniki
- Meneame
- Blogger
- Amazon
- Yahoo Mail
- Gmail
- AOL
- Newsvine
- HackerNews
- Evernote
- MySpace
- Mail.ru
- Viadeo
- Line
- Comments
- Yummly
- SMS
- Viber
- Telegram
- Subscribe
- Skype
- Facebook Messenger
- Kakao
- LiveJournal
- Yammer
- Edgar
- Fintel
- Mix
- Instapaper
- Copy Link
Posted: 6 September 2011 | Marina Witthuhn, Jörg Hinrichs and Zeynep Atamer, Universität Hohenheim, Institute of Food Science and Biotechnology, Department of Dairy Science and Technology | No comments yet
In order to obtain safe dairy products with a long shelf-life, heating processes have been designed to ensure the necessary inactivation of the indigenous microbial flora. One of the methods is the ultra-high temperature (UHT) processing of milk which has become widespread since the implementation of aseptic packaging processes1. Nowadays, this method is not only used for milk, but it is also applied to a whole range of dairy products with different microbial, physical and chemical properties. Therefore, new challenges for the manufacturers arise as these products require a different treatment to guarantee the safety and quality. UHT processing in general as well as emerging trends and challenges in processing of milk and milk products will be discussed in this article.
UHT processing of milk generally refers to processes that are conducted at 135 to 150°C for 10 to one seconds1,2. In production plants working with direct steam injection, even higher temperatures of 150 to 152°C are used for up to 13 seconds3. In contrast to this, sterilisation of milk is realised at temperatures varying from 109 to 120°C for 40 to 20 minutes2. These two processes are designed in such a way that the destruction of microorganisms of importance (bacterial spores) is the same in both cases as this is essential for the sterility of the final product. However, the effects on vitamins and other substances (e.g., lactose, amino acids) can differ greatly3. Nowadays, with the emerging application of UHT treatments to dairy products other than milk, such as products containing functional ingredients (e.g., infant food), new processing conditions have to be defined.
In order to obtain safe dairy products with a long shelf-life, heating processes have been designed to ensure the necessary inactivation of the indigenous microbial flora. One of the methods is the ultra-high temperature (UHT) processing of milk which has become widespread since the implementation of aseptic packaging processes1. Nowadays, this method is not only used for milk, but it is also applied to a whole range of dairy products with different microbial, physical and chemical properties. Therefore, new challenges for the manufacturers arise as these products require a different treatment to guarantee the safety and quality. UHT processing in general as well as emerging trends and challenges in processing of milk and milk products will be discussed in this article. UHT processing of milk generally refers to processes that are conducted at 135 to 150°C for 10 to one seconds1,2. In production plants working with direct steam injection, even higher temperatures of 150 to 152°C are used for up to 13 seconds3. In contrast to this, sterilisation of milk is realised at temperatures varying from 109 to 120°C for 40 to 20 minutes2. These two processes are designed in such a way that the destruction of microorganisms of importance (bacterial spores) is the same in both cases as this is essential for the sterility of the final product. However, the effects on vitamins and other substances (e.g., lactose, amino acids) can differ greatly3. Nowadays, with the emerging application of UHT treatments to dairy products other than milk, such as products containing functional ingredients (e.g., infant food), new processing conditions have to be defined.
In order to obtain safe dairy products with a long shelf-life, heating processes have been designed to ensure the necessary inactivation of the indigenous microbial flora. One of the methods is the ultra-high temperature (UHT) processing of milk which has become widespread since the implementation of aseptic packaging processes1. Nowadays, this method is not only used for milk, but it is also applied to a whole range of dairy products with different microbial, physical and chemical properties. Therefore, new challenges for the manufacturers arise as these products require a different treatment to guarantee the safety and quality. UHT processing in general as well as emerging trends and challenges in processing of milk and milk products will be discussed in this article.
UHT processing of milk generally refers to processes that are conducted at 135 to 150°C for 10 to one seconds1,2. In production plants working with direct steam injection, even higher temperatures of 150 to 152°C are used for up to 13 seconds3. In contrast to this, sterilisation of milk is realised at temperatures varying from 109 to 120°C for 40 to 20 minutes2. These two processes are designed in such a way that the destruction of microorganisms of importance (bacterial spores) is the same in both cases as this is essential for the sterility of the final product. However, the effects on vitamins and other substances (e.g., lactose, amino acids) can differ greatly3. Nowadays, with the emerging application of UHT treatments to dairy products other than milk, such as products containing functional ingredients (e.g., infant food), new processing conditions have to be defined.
As mentioned above, the aim of UHT processing of milk and other dairy products is to obtain products with a long shelf-life. However, complete sterilisation of these products cannot be achieved in practice; they still contain a certain level of resistant microorganisms1. Therefore, it is common practice to define an accepted level of non-sterility p in order to produce ‘commercially sterile’ products. For example, p = 10-4 means that one non-sterile package per 10 000 produced ones is tolerated. When designing UHT processes, inactivation of thermophilic bacterial spores such as spores of Geobacillus stearothermophilus is mainly considered since they are capable of surviving the UHT heating. For the calculation of the required inactivation effect B* to obtain commercially sterile products with a predefined level of non-sterile products, the following equation is used4:
B*: required inactivation effect; N0: initial spore count of the raw material; p: accepted level of non-sterility; V: package volume
As can be seen from Equation 1, the necessary inactivation effect depends amongst others on the package size. If a volume of one litre (V = 103 mL) UHT-milk and an initial spore contamination level of approximately 102 spores per mL were taken into account, a 9 log inactivation of thermophilic spores should be aimed at in a typical UHT-milk process. This level was defined by Kessler2 as the minimal inactivation effect required for manufacturing commercially sterile products and it represents the lower limit of heat treatments for UHT and other sterilisation processes. This required minimal inactivation of thermophilic spores can be obtained with several temperature-time combinations, which form the line (B* = 9) shown in Figure 1. Additionally, an upper limit for UHT processes was given by Kessler2. The author defined it as the processing conditions which result in less than three per cent loss of thiamine, a thermolabile vitamin present in milk. The line of equal effect for the loss of thiamine is likewise illustrated in Figure 1. Any temperature-time combination in the region of sterilisation (Figure 1) also results in more than 9 log reduction of thermophilic spores; however, the chemical changes e.g., browning, loss of thiamine, would be more severe than in a UHT treatment.
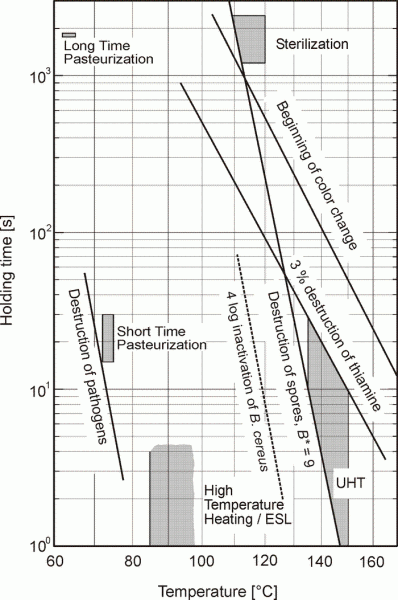
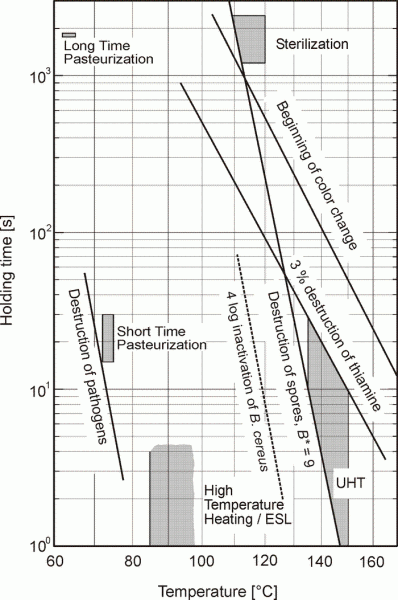
Figure 1 Temperature-time diagram for milk heat treatments with lines of equal effect for B* = 9 log and a three per cent loss of thiamine (adapted from Kessler2)
Emerging spores of importance for dairy processing
Ultra-high temperature heating processes are not only applied for the sterilisation of milk, but also for the production of milk-based beverages such as flavoured milks e.g., chocolate milk, and sports drinks, as well as for functional foods, processed cheeses and milk concentrates prior to drying1,5. Today, there is already a wide variety of milk beverages and formulas with long shelflife, in which special, partly imported milk fractions, exotic fruits, functional additives or stabilisers are added. In doing so, thermoresistant spore species may enter the product to be preserved. The use of exotic and imported food ingredients in new products, however, creates new niches and risks of introducing new unknown heat-stable spore-formers into the production process. In addition, the expanding worldwide trade with products of long shelf-life can lead to the distribution of very heat-stable spores.
When very heat-resistant spores are present in the raw material, the temperature-time combination of the UHT process might have to be adapted accordingly. Therefore, there is an increasing necessity to identify and determine the heat resistance of spores which are brought into the product chain via raw materials and ingredients. Studies have already been conducted to determine the spore load of typical ingredients used in milk processing. In Table 1, some commonly used ingredients in dairy processing and their spore loads are listed. It can be seen that ingredients contain sometimes high numbers of spores. This does not pose problems if the spores are completely inactivated during the applied treatment; however, some spores have a high survival potential showing extreme heat stability (e.g., Scheldeman et al.6)
Table 1: Examples of ingredients commonly used in dairy processing with spore load
Ingredient |
Spore count [cfu g-1 or mL-1] |
Genera |
Source |
Milk powder |
Up to 104 |
Bacillus, Anoxybacillus, Geobacillus |
[7] |
Cocoa powder |
Up to 104 |
Bacillus, Geobacillus |
[8] |
Herbs |
Up to 105 |
Not determined |
[9] |
Spices |
Up to 107 |
Bacillus, Geobacillus |
[10] |
Raw milk |
Up to 102 |
Different genera, including Bacillus |
[11] |
In own studies, the heat resistance of emerging spores of strains isolated from different dairy products and ingredients was determined. This was performed in order to gain a database of the thermal stability of the spores introduced via ingredients into the final product. Firstly, the strains were isolated and characterised. Spore suspensions of the isolated strains were produced. Then, a heat treatment was performed. In Figure 2, the results for the inactivation of different species isolated from various food sources after a treatment of 30 minutes at 120°C are illustrated. The upper end of the bar signifies the initial spore count N0 before the heat treatment; the lower end represents the spore count after the heat treatment. On the x-axis, the food sources of the isolates are given. As can be seen in Figure 2, highly heat-resistant spore-formers were found in a variety of food products. Ingredients such as cocoa powder or spices, which were shown to contain highly heat-resistant spores (Figure 2) as well as high amounts of spores (Table 1) as determined in recent studies, are often used for the manufacturing of dairy products. Therefore, when intending to use these ingredients for the production of long-life products, attention has to be paid to their microbial quality. If necessary, the process has to be adapted according to the stability of the newly introduced spores.
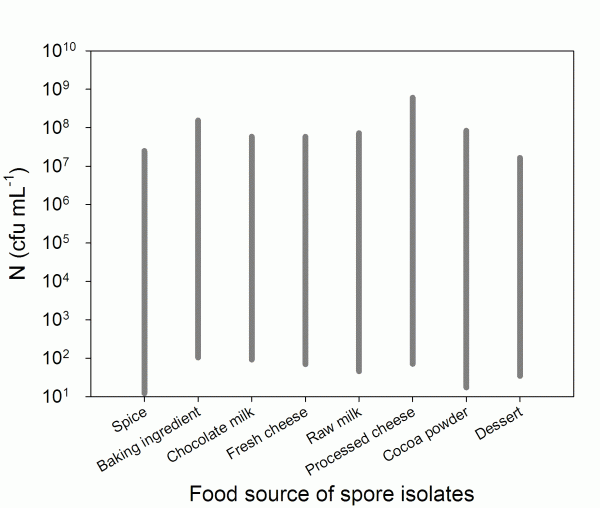
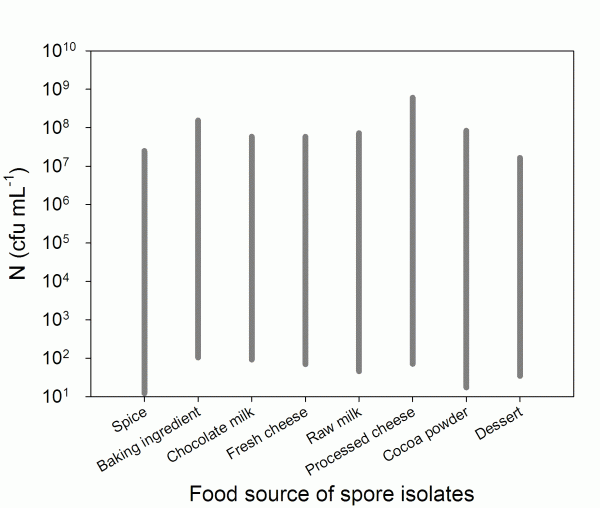
Figure 2 Inactivation of spores isolated from different food sources after a treatment for 30 minutes at 120°C in phosphate buffer. The upper limit of the bar signifies the initial spore N0 before the heat treatment; the lower limit represents the spore count after the heat treatment
When the inactivation kinetics of the emerging spores are known, a line of equal effect for a 9 log inactivation of the corresponding spore similar to the one shown in Figure 1 can be calculated and presented in the same diagram. In doing so, improved processing conditions can easily be defined.
Activation of spores – a hazard to the design of safe processes
As demonstrated before, the initial spore count of the raw material is crucial for the sterility of the final product and the process is designed according to the initial concentration of spores (Equation 1). But the question is how exact the method to determine the initial spore count is. Spores usually require an activation step, e.g., with a sublethal heat treatment, to trigger germination. But as the temperatures needed for this heat activation can differ greatly between the different species12, not all the spores present in the product might be detected when determining the initial spore count.
To determine the amount of bacterial spores in food samples, the standard procedures usually suggest heating of the product at 80°C for 10 minutes before analysis (e.g., VDLUFA13). This heating step aims at killing vegetative cells. Some spores, however, require higher activation temperatures to start germinating. The activation temperature depends very much on the strain itself. It was shown that the higher the wet-heat resistance of the spores is, the higher the optimal activation temperature is14. For spores of Geobacillus stearothermophilus, an activation temperature of 121°C was suggested12. In food processing, this means that when determining the initial spore load of ingredients, not all the spores might be detected because they have not been activated and cannot therefore start germinating. This may lead to an underestimation of the initial spore load N0, which plays an important role in defining the processing conditions for the production of commercially sterile products. The heat activation of spores has also been observed in our recent studies and this observation is demonstrated in Figure 3. Inactivation curves of spores of Geobacillus stearothermophilus, a thermophilic species also occurring in food products, were determined. The initial spore count N0 was approximately 107 cfu mL-1, whereas after the heat treatments, the spore count N increased up to 109 cfu mL-1. An increase of almost 2 log units in the spore count due to heat activation was observed. Consequently, the presence of such spores in the ingredients and the usage of spore counts before the activation of these spores as the initial load would result in erroneous assumptions and interpretations for the manufacturers and the spores that remain inactivated after the UHT processing can then start growing in the food product. As a conclusion, more attention has to be paid to the correct determination of the initial spore count as this has consequences for the whole production process.
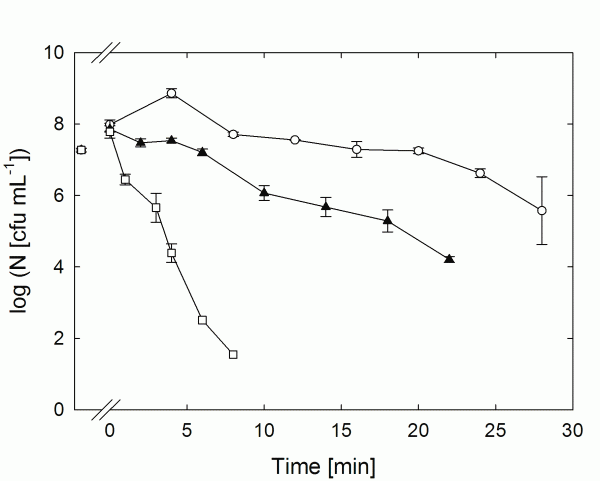
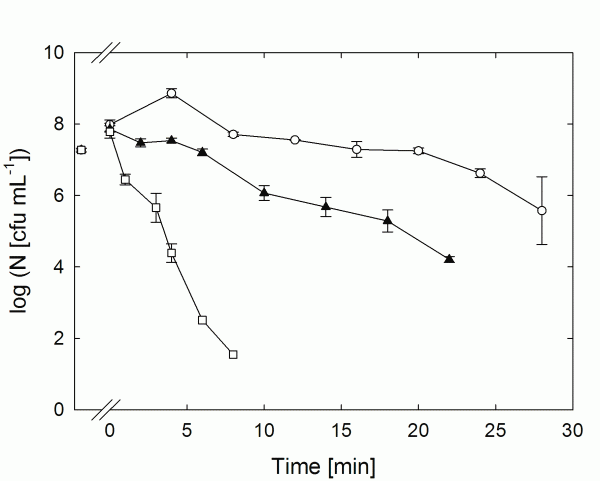
Figure 3 Inactivation curves of Geobacillus stearothermophilus at 120°C (●), 125°C (▲) and 130°C (■) with activation shoulder at 120°C
UHT processing of dairy products other than milk
More and more dairy products other than milk are also treated using UHT processes. As these dairy products have different microbial properties, which was discussed before, as well as different physical and chemical properties, the processes have to be adjusted based on the product properties. The challenges that arise with these new products include the minimisation of fouling and gelation during the treatment due to high protein or calcium contents. When functional foods are processed at UHT conditions, the stability of the bioactives has to be maintained during processing and storage1.
One example of a milk product with a high content of bioactive compounds is infant food. On the one hand, microorganisms have to be inactivated in order to assure the safety of the infant food. On the other hand, the loss of functionality such as the destruction of vitamins, the denaturation of proteins and the formation of off-flavours has to be kept at a minimum15. In this case, the application of ultra-high temperatures (> 150°C) and ultra-short residence times (<0.2 seconds) might offer new opportunities regarding the microbial inactivation while preserving the product quality16. This process is a recent invention called innovative steam injection (ISI) using direct heating. As this method allows very short heating times, the heat load for the product is reduced. Hence, functional ingredients are only slightly affected while the required destruction of bacterial spores as well as of indigenous milk proteins like plasmin is achieved16.
Another challenge to the manufacturer is when milk concentrates are treated in UHT processes. Several problems arise while heating: the reduced water activity due to the high dry matter content which enhances the heat stability of the spores, the high viscosity of the concentrate which demands new processing solutions such as scraped-surface heat exchangers and the high dry matter content in the product which promotes aggregation and gelling during processing and storage2,5. Hinrichs5 showed that the heat stability of a concentrate at 140°C depends amongst others on the mineral content. This was demonstrated by comparing concentrates produced using ultrafiltration, nanofiltration and reverse osmosis. The higher the mineral content of the concentrate was, the lower the heat stability and the heat coagulation during heating were. The most heat-stable concentrates were reported to be those produced by means of ultrafiltration. These concentrates had the lowest mineral content among the examined concentrates. The described relationship between the heat stability which is expressed as relative heat coagulation time and the mineral content of a whole milk concentrate is illustrated in Figure 4. From these results, it can be concluded that the significant influence of the mineral content of the milk concentrates on the heat stability should be considered when designing the processes for treating milk concentrates at ultra-high temperatures. But not only the heat stability of the concentrate needs to be taken into account. An additional problem when heating concentrates with the aim of spore inactivation is their reduced water activity as spores are more heat resistant at low aw-values2. Behringer17 examined the in – activation of Bacillus licheniformis spores in skim milk and skim milk concentrate. He found that the inactivation rate is slower in the concentrate (Figure 5). Adjustment of the pH of the concentrate to the pH of skim milk led to an even higher thermostability. Consequently, inactivation kinetics of spores that were determined in milk might not be sufficient for the design of UHT processes for concentrates. Inactivation kinetics obtained in milk concentrate should rather be used.
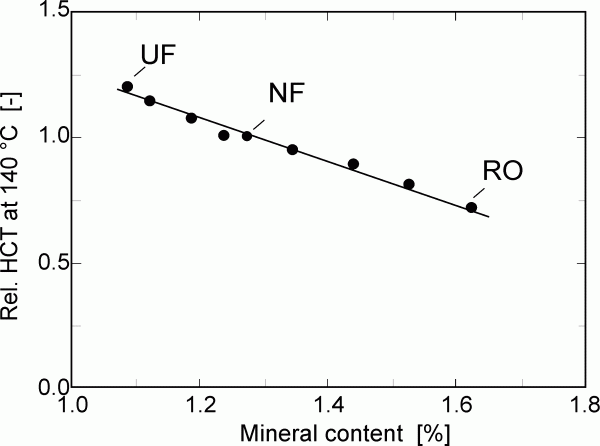
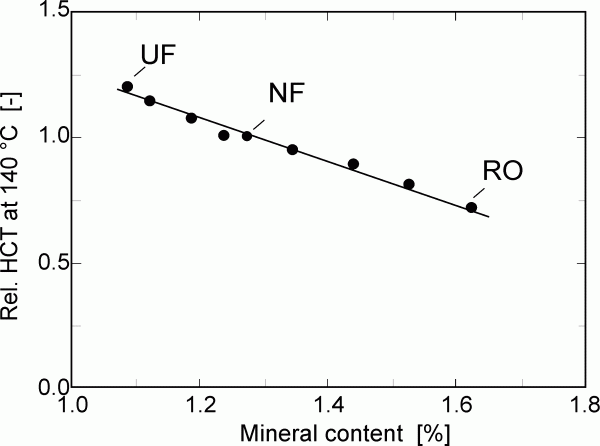
Figure 4 Relationship between the relative heat coagulation time (HCT) and the mineral content of a whole milk concentrate (3.8 per cent fat)5. UF: ultrafiltration, NF: nanofiltration, RO: reverse osmosis. Points in between are mixtures of the concentrates
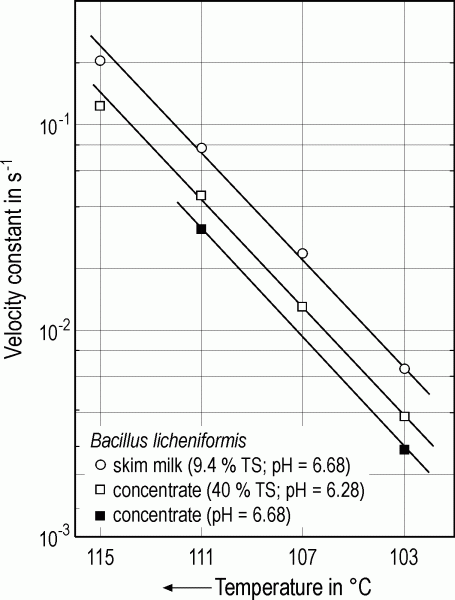
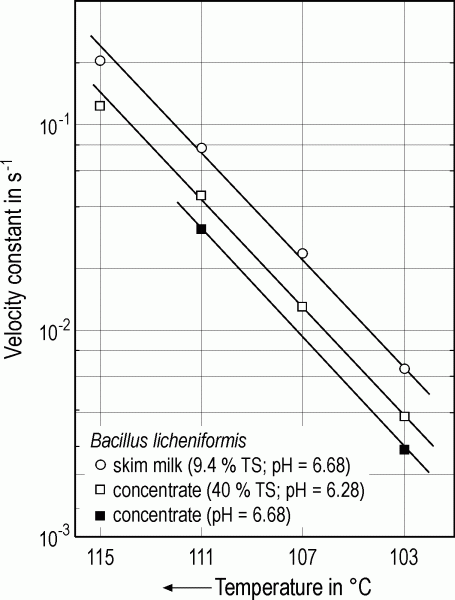
Figure 5 Effect of temperature on the velocity constant of the destruction of Bacillus licheniformis spores in skim milk and skim milk concentrate (adapted from Behringer17)
Conclusion
UHT processing is of growing importance for the production of dairy products. Today, there is a wide variety of milk beverages and formulas with long shelf-life, the production of which carries the risk of introducing unknown heat stable spores due to the use of exotic and imported new food ingredients. Several factors contribute to the safety and quality of the final product which has varying microbial, chemical and physical properties. In order to produce products of high quality and with a long shelflife, the properties of the products such as milk concentrates, functional foods or processed cheeses as well as their behaviour and stability during the heating process have to be known. Then, the UHT heating process can be designed according to the defined characteristics of the product to be heated, which leads to achieving the desired results.
References
1. Deeth, H., Improving UHT processing and UHT milk products, in Improving the safety and quality of milk, M.W. Griffiths, Editor. 2010, Cambridge, UK: Woodhead Publishing Limited
2. Kessler, H.G., Food and Bio Process Engineering – Dairy Technology. 2002, München: Verlag A. Kessler
3. Cattaneo, S., Masotti, F. and Pellegrino, L., Effects of overprocessing on heat damage of UHT milk. European Food Research and Technology, 2008. 226(5): 1099-1106
4. Hinrichs, J., Buck, H. and Hauser, G., Sterilisation und Sterildesign, in Bioprozesstechnik, H. Chmiel, Editor. 2011, Heidelberg: Spektrum Akademischer Verlag
5. Hinrichs, J., UHT processed milk concentrates. Lait, 2000. 80(1): 15-23
6. Scheldeman, P., Pil, A., Herman, L., De Vos, P. and Heyndrickx, M., Incidence and diversity of potentially highly heat-resistant spores isolated at dairy farms. Applied and Environmental Microbiology, 2005. 71(3): 1480-1494
7. Rückert, A., Ronimus, R.S. and Morgan, H.W., A RAPDbased survey of thermophilic bacilli in milk powders from different countries. International Journal of Food Microbiology, 2004. 96(3): 263-272
8. Lima, L.J.R., Kamphuis, H.J., Nout, M.J.R. and Zwietering, M.H., Microbiota of cocoa powder with particular reference to aerobic thermoresistant spore-formers. Food Microbiology, 2011. 28(3): 573-582
9. Witkowska, A.M., Hickey, D.K., Alonso-Gomez, M. and Wilkinson, M.G., The microbiological quality of commercial herb and spice preparations used in the formulation of a chicken supreme ready meal and microbial survival following a simulated industrial heating process. Food Control, 2011. 22(3-4): 616-625
10. Oomes, S.J.C.M., van Zuijlen, A.C.M., Hehenkamp, J.O., Witsenboer, H., van der Vossen, J.M.B.M. and Brul, S., The characterisation of Bacillus spores occurring in the manufacturing of (low acid) canned products. International Journal of Food Microbiology, 2007. 120(1- 2): 85-94
11. Coorevits, A., De Jonghe, V., Vandroemme, J., Reekmans, R., Heyrman, J., Messens, W., De Vos, P. and Heyndrickx, M., Comparative analysis of the diversity of aerobic spore-forming bacteria in raw milk from organic and conventional dairy farms. Systematic and Applied Microbiology, 2008. 31(2): 126-140
12. Russel, A.D., The Destruction of Bacterial Spores. 1982, London: Academic Press.
13. VDLUFA, Verband Deutscher Landwirtschaftlicher Untersuchungs- und Forschungsanstalten Methodenbuch Band VI. 1985, Darmstadt: VDLUFAVerlag
14. Ghosh, S., Zhang, P., Li, Y.Q. and Setlow, P., Superdormant spores of Bacillus species have elevated wet-heat resistance and temperature requirements for heat activation. Journal of Bacteriology, 2009. 191(18): 5584-5591
15. Floris, R., Lambers, T., Alting, A. and Kiers, J., Trends in infant formulas: a dairy perspective, in Improving the safety and quality of milk, M.W. Griffiths, Editor. 2010, Cambridge, UK: Woodhead Publishing Limited
16. van Asselt, A.J., Sweere, A.P.J., Rollema, H.S. and de Jong, P., Extreme high-temperature treatment of milk with respect to plasmin inactivation. International Dairy Journal, 2008. 18(5): 531-538
17. Behringer, R., Über das Absterbeverhalten von Bacillus- Sporen in Milch- und Milchkonzentraten. 1989, Technische Universität München
Marina Witthuhn is currently working as a research assistant at the University of Hohenheim (Germany) in the department of Dairy Science and Technology. Her research focuses on modelling and prediction of quality and safety of food products and processes. Ms. Witthuhn has a degree in Food Engineering of the University of Hohenheim.
Prof. Dr.-Ing. Jörg Hinrichs has been Head of the department of Dairy Science and Technology at the University of Hohenheim, Germany since 2001. His scientific career began at the Technical University of Munich, where he finished his Habilitation in 2000. Mr. Hinrichs is interested in micro- and macro-structure of dairy products, innovative processes and process safety and quality.
Zeynep Atamer is a research assistant at the University of Hohenheim, Germany. Mrs. Atamer has a BS in Food Engineering, together with a minor degree in Environmental Engineering, from the Middle East Technical University, Turkey. She received her MS in Environmental Protection and Food Production from the University of Hohenheim.