Emulsifiers in food
- Like
- Digg
- Del
- Tumblr
- VKontakte
- Buffer
- Love This
- Odnoklassniki
- Meneame
- Blogger
- Amazon
- Yahoo Mail
- Gmail
- AOL
- Newsvine
- HackerNews
- Evernote
- MySpace
- Mail.ru
- Viadeo
- Line
- Comments
- Yummly
- SMS
- Viber
- Telegram
- Subscribe
- Skype
- Facebook Messenger
- Kakao
- LiveJournal
- Yammer
- Edgar
- Fintel
- Mix
- Instapaper
- Copy Link
Posted: 4 January 2012 | John Coupland, Professor of Food Science, Penn State University | No comments yet
Very often, the most important ingredients in food are those present in the smallest quantities. Flavours, phytochemicals and micronutrients compounds may only be added at parts per million levels but their presence is essential to determining the functional properties of the food. While these molecules differ in both structure and function, many share common features. First, they are very poorly water-soluble, typically less than a few grams per litre, but are often much more soluble in oils. The partitioning between a lipid phase and water is given by a partition coefficient, the ratio of concentrations in each phase at equilibrium.
For many of the molecules of interest, the partition coefficient is in the order one thousand in favour of the lipid phase. Second, they are readily destroyed by chemical oxidation and/or acid hydrolysis. Interestingly, the metals that catalyse their oxidation and the acids that cause hydrolysis are much more soluble in water but much less soluble in oil. Finally, they are expensive; flavours in particular can be huge contributors to the overall cost of the product. This last point means that there is a commercial pressure to ensure all of the molecules added to the food are active within the food. To begin to solve this problem we need to consider how the molecules are distributed within the food.
Very often, the most important ingredients in food are those present in the smallest quantities. Flavours, phytochemicals and micronutrients compounds may only be added at parts per million levels but their presence is essential to determining the functional properties of the food. While these molecules differ in both structure and function, many share common features. First, they are very poorly water-soluble, typically less than a few grams per litre, but are often much more soluble in oils. The partitioning between a lipid phase and water is given by a partition coefficient, the ratio of concentrations in each phase at equilibrium. For many of the molecules of interest, the partition coefficient is in the order one thousand in favour of the lipid phase. Second, they are readily destroyed by chemical oxidation and/or acid hydrolysis. Interestingly, the metals that catalyse their oxidation and the acids that cause hydrolysis are much more soluble in water but much less soluble in oil. Finally, they are expensive; flavours in particular can be huge contributors to the overall cost of the product. This last point means that there is a commercial pressure to ensure all of the molecules added to the food are active within the food. To begin to solve this problem we need to consider how the molecules are distributed within the food.
Very often, the most important ingredients in food are those present in the smallest quantities. Flavours, phytochemicals and micronutrients compounds may only be added at parts per million levels but their presence is essential to determining the functional properties of the food. While these molecules differ in both structure and function, many share common features. First, they are very poorly water-soluble, typically less than a few grams per litre, but are often much more soluble in oils. The partitioning between a lipid phase and water is given by a partition coefficient, the ratio of concentrations in each phase at equilibrium.
For many of the molecules of interest, the partition coefficient is in the order one thousand in favour of the lipid phase. Second, they are readily destroyed by chemical oxidation and/or acid hydrolysis. Interestingly, the metals that catalyse their oxidation and the acids that cause hydrolysis are much more soluble in water but much less soluble in oil. Finally, they are expensive; flavours in particular can be huge contributors to the overall cost of the product. This last point means that there is a commercial pressure to ensure all of the molecules added to the food are active within the food. To begin to solve this problem we need to consider how the molecules are distributed within the food.
Take a simple lipophilic food ingredient, say eugenol (Figure 1), and add it to the simplest of foods – water. A small amount will dissolve, but after the solubility limit is exceeded any excess will either form pools or clumps of undissolved material (Figure 2 – fat free). The process of dissolution may be very slow and in many practical cases ‘it won’t dissolve’ means ‘you haven’t given it enough time to dissolve!’ If the goal is to measure the solubility limit, it is far easier to allow an excess of solute to come to equilibrium with the water over days or weeks than filtering off the undissolved material and measuring the concentration of the solution.
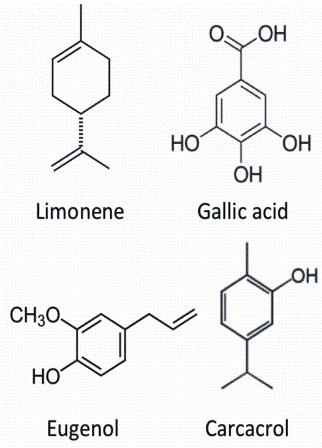
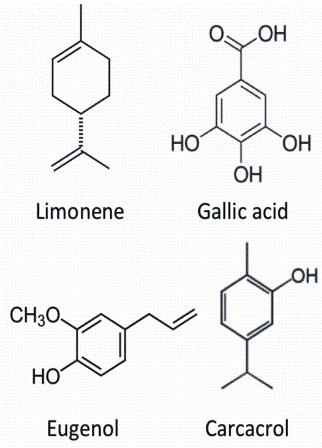
FIGURE 1 Chemical structure of some lipophilic food additive
The dissolved eugenol will be vulnerable to reaction with acids and metals so will tend to degrade over time, lowering the concentra – tion of the solution. As this happens, more of the undissolved ingredient can dissolve to maintain the concentration at the solubility limit. However, dissolution takes time; molecules would have to detach from the surface of the clump or pool of undissolved ingredient then diffuse through the food to even out the aqueous concentration. This can easily lead to ‘dead spots’ with no active ingredient at all. While this might not matter for a drink shaken before consumption, it could have real food safety consequences if the molecule of interest was an antimicrobial compound. There needs to be a better way to keep the molecules of interest homogeneously distributed around the food. One obvious solution is to emulsify them in oil.
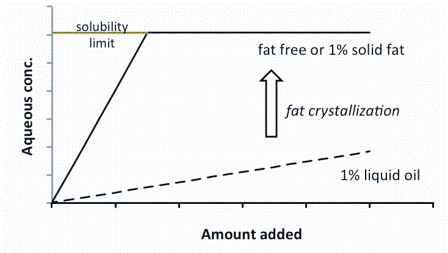
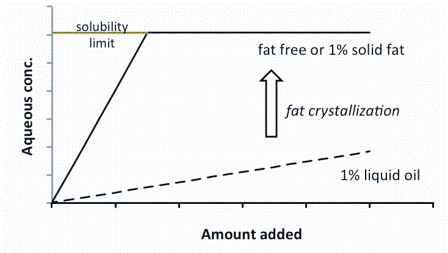
FIGURE 2Aqueous concentration of a lipophilic ingredient as a function of the amount added and in the presence and absence of a small amount of emulsified oil (one per cent). Crystallisation of the fat removes its effectiveness as a reservoir for the ingredient and it behaves effectively the same as the fat free product
The ingredient can be dissolved in liquid oil then homogenised with an appropriate emulsifier system to produce a physically stable dispersion that is readily dilutable in aqueous food. The presence of the oil allows much more of the molecule of interest to be dispersed within the food but lowers the aqueous concentration due to the partitioning effect. Figure 2 shows the effect of adding increasing amounts of a model compound to an aqueous food in the presence and absence of one per cent oil. In the absence of oil, the aqueous concentration increases rapidly but quickly reaches a plateau at the solubility limit. In the presence of the oil, the aqueous concentration increases, but much more slowly as most of the added molecules have partitioned into the oil phase (remember even though the amount of lipid is low compared to the amount of water, the concentration of our ingredient is 1000 times greater in the lipid phase). However, by carrying the ingredient in an emulsion droplet, the food technologist has achieved much greater control. First, the droplets are intimately mixed within the food so the diffusion paths from the droplet are much smaller and the aqueous phase will remain relatively homogeneous with respect to the ingredient. Secondly, the properties of the interface separating the droplet from the aqueous phase can be tuned by selecting the emulsifier system.
Emulsifiers are amphiphilic molecules (i.e., both water-loving and water-hating) so they tend to accumulate at the interface between the oil droplets and water in an emulsion. Once there, emulsifiers both lower the interfacial tension and provide a barrier stopping the droplets from coalescing. In foods, most emulsifiers are proteins (e.g., casein, whey, gelatin) although small molecule surfactants (e.g., lecithin extracted from soy or egg or synthetic molecules such as Spans and Tweens) are also used. Emulsifiers are essential to producing an emulsion that will remain stable over the lifetime of the product. However, when the emulsion is being used as a delivery system for small molecules it can have a secondary role protecting them from reaction.
As we noted earlier, the ingredients of interest tend to partition into the oil phase while the things they react with (e.g., metal ions, acids) are aqueous. The emulsifier can act as a barrier keeping the two reactive materials apart. While the physical strength and thickness of the barrier may play a role, it appears the dominant effects are electrostatic – that is they depend on the repulsion between like charges. For example, Warharo, McClements and Decker1 looked at the rate of oxidation of w3 fatty acids in a water-in-oil emulsion. While oxygen is present throughout the emulsion, it can only react significantly with the lipid in the presence of an iron catalyst. When the emulsifier carried a negative charge, it attracted the iron ions to the surface and the emulsion quickly went rancid while on the other hand when the surface was positive the iron could not approach the surface and the lipid was protected. Understanding the relations between emulsion structure and ingredient functionality is a breakthrough but could it be extended? Is it possible to further change the properties of the emulsion to affect how the ingredient will behave?
One obvious target would be controlled release. If a flavour for example could be protected by an emulsion during manufacture and storage yet released at time of consumption, it would be possible to use less of the expensive ingredient in the first place. In this case varying the properties of the emulsifier is probably not going to be a helpful. To limit the movement of the small molecule out of the droplet the surface layer would have to be very thick and dense and most structures available are not. A more promising tool would be to change the volume of liquid oil present.
Clearly, changing the gross composition of a food after manufacture is impossible but there is a work-around. Oils can crystallise to different solid fat contents depending on their chemical composition and temperature. Most of the evidence points to the fat crystals being incompatible with most of the added ingredients we are interested in. What this means is when the oil crystallises, it can no longer act as a solvent for the ingredient. In terms of Figure 2, if the oil in the oil containing sample suddenly crystallised, the aqueous concentration would increase to equal that of the fat-free sample. The undissolved ingredient would probably remain stuck to the surface of the crystalline particle so would still be intimately mixed with the food. The change occurs very rapidly and crystallisation could be triggered by a change in temperature or allowed to occur spontaneously over time.
Crystalline emulsion droplets used as a carrier for lipophilic food ingredients are known in the pharmaceuticals field as solid lipid nanoparticles (SLN)2. SLN have been formulated using very high melting fats and food grade emulsifiers and have been shown to make the encapsulated ingredient active (i.e., flavours more volatile3, carotenoids more prone to oxidation4) than a similar liquid oil droplet. The technology is real; the challenge is to find applications where rapidly adjustable functionality for small molecule ingredients can be used to confer some practical benefit to the food.
References
1. Waraho T, McClements DJ, Decker EA 2011. Mechanisms of lipid oxidation in food dispersions, Trends in Food Science and Technology, 22, 3-13
2. Bunjes, H. 2010. Lipid nanoparticles for the delivery of poorly water-soluble drugs.Journal of Pharmacy and Pharmacology, 62, 1637-1645
3. Ghosh S, Peterson DG and Coupland, JN. 2006. Effects of droplet crystallization and melting on the aroma release properties of a model oil-in-water emulsion. Journal of the Agricultural and Food Chemistry, 54, 1829-1837
4. Helgason T, Awad TS, Kristbergsson K, Decker EA , McClements DJ, Weiss J. 2009. Impact of Surfactant Properties on Oxidative Stability of beta-Carotene Encapsulated within Solid Lipid Nanoparticles, Journal of Agricultural and Food Chemistry, 57, 8033-8044