Unravelling acid whey processing by understanding lactose crystallisation
- Like
- Digg
- Del
- Tumblr
- VKontakte
- Buffer
- Love This
- Odnoklassniki
- Meneame
- Blogger
- Amazon
- Yahoo Mail
- Gmail
- AOL
- Newsvine
- HackerNews
- Evernote
- MySpace
- Mail.ru
- Viadeo
- Line
- Comments
- Yummly
- SMS
- Viber
- Telegram
- Subscribe
- Skype
- Facebook Messenger
- Kakao
- LiveJournal
- Yammer
- Edgar
- Fintel
- Mix
- Instapaper
- Copy Link
Posted: 16 August 2016 | Coen Akkerman - NIZO Food Research B.V., Marja W. Kanning - NIZO Food Research B.V., Thom Huppertz - NIZO Food Research B.V. | No comments yet
Nowadays acid whey typically refers to the ever expanding amounts of whey originating from Greek style yoghurt. However, not every acid whey is the same. As lactose crystallisation in acid whey is complex and affected by many factors, including the presence of lactic acid, minerals and proteins, it is more challenging to prepare non-hydroscopic powders from acid whey than from sweet whey. Improvement of whey (permeate) powders of industrially available inhomogeneous whey streams requires understanding of the complex interactions of whey composition and applied processing.
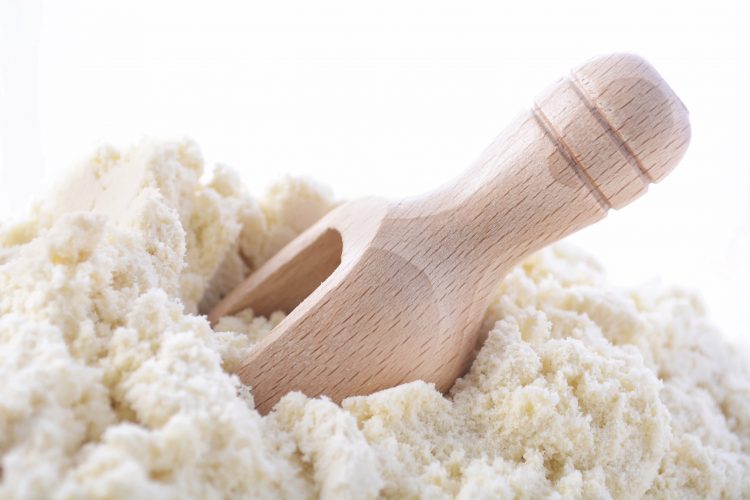
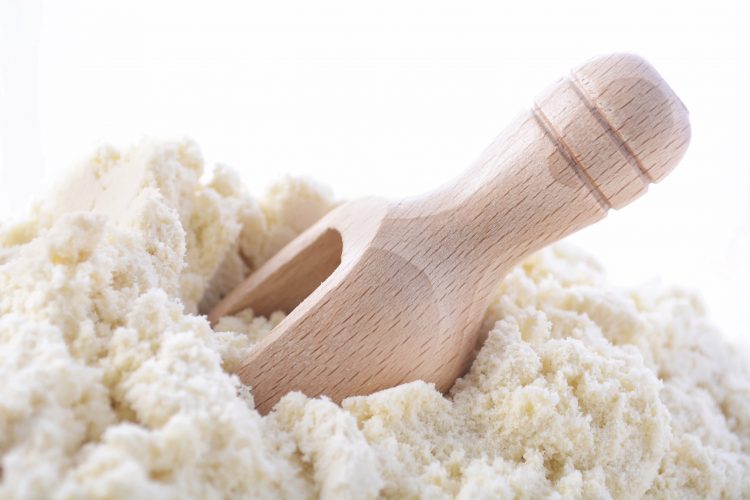
The dairy industry produces various high lactose products and ingredients. Drying of high-lactose products requires careful processing, as these products can easily cause difficulties due to stickiness, hygroscopicity, etc. Normal skimmed milk powder has a lactose content of >50%. Even higher levels of lactose are present in whey powder (~61%),1 permeate powder (~80%) and lactose (>98%). Pre-crystallisation of lactose is often carried out to make non-hygroscopic powders, by minimising the presence of the hygroscopic amorphous lactose. Pre-crystallisation typically involves concentration followed by controlled cooling. These processes for lactose, permeate and whey powder production are well established and widely applied industrially. However, considerably less knowledge is available for acid whey types, which contain other compounds that can strongly affect crystallisation behaviour; in particular, lactic acid has a strong effect on lactose crystallisation behaviour. Lactic acid is present only in small amounts in whey derived from cheese manufacture or from casein production using mineral acids, but in much larger amounts in acid whey from fermented dairy products. This can impair crystallisation behaviour.
Acid whey composition
The volume of acid whey available is growing significantly as Greek and Greek-style yoghurt products are becoming more and more popular. Whey streams from, for example, cottage cheese, quark, tvarog and casein production further contribute to the volume of acid whey. These different whey streams differ considerably in composition. To illustrate the differences in whey composition; whey from the manufacture of cheese curd, casein, quark and yoghurt were produced on a pilot plant scale from a batch of skimmed milk, and the various types of whey showed clear differences in composition, as seen in Table 1.
All acid whey products consist of ~6% dry matter, of which the majority is lactose. The protein concentration of acid whey from yoghurt or quark products is very low due to the fact that most whey proteins are denatured and retained in the product matrix; in contrast, protein concentration from cheese or casein manufacture contains virtually all the whey protein that was present in the milk. Compared to cheese whey, acid whey contains a large amount of acid and an increased amount of ash (in particular Ca and P). Whey from acid casein production differs in mineral composition, depending on the mineral acid used for acidification (HCl or H2SO4). In the case of whey from yoghurt manufacture, lactic acid is present. Owing to the fact that lactic acid is a weak acid, titratable acidity of acid whey from yoghurt and quark manufacture is higher than that of whey from acid casein manufacture. Furthermore, depending on the culture used for fermentation, galactose may or may not be present in acid whey, as highlighted by differences in galactose content between whey from yoghurt and quark manufacture (Table 1). All these compositional differences should be considered in relation to lactose crystallisation to enable preparation of non-hygroscopic powders.
Factors affecting lactose crystallisation
Lactose crystallisation consists of a series of steps that strongly depend on the composition and process conditions (Figure 1). To achieve lactose crystallisation, lactose solubility needs to be exceeded enough for nucleation to occur; once stable nuclei are formed, they can grow to form lactose crystals. Lactose solubility, nucleation and crystal growth can be affected by various non-lactose whey constituents, eg, lactic acid and minerals. As a result, the degree of crystallinity, the number of crystals as well as the size and shape are affected by the medium in which crystallisation is carried out.
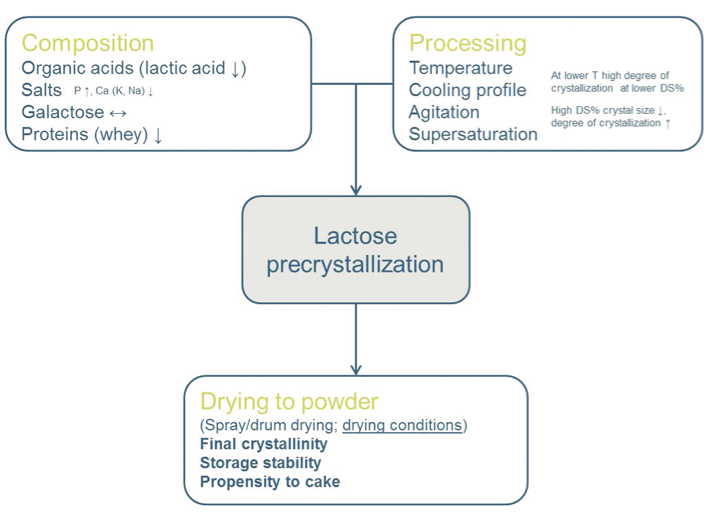
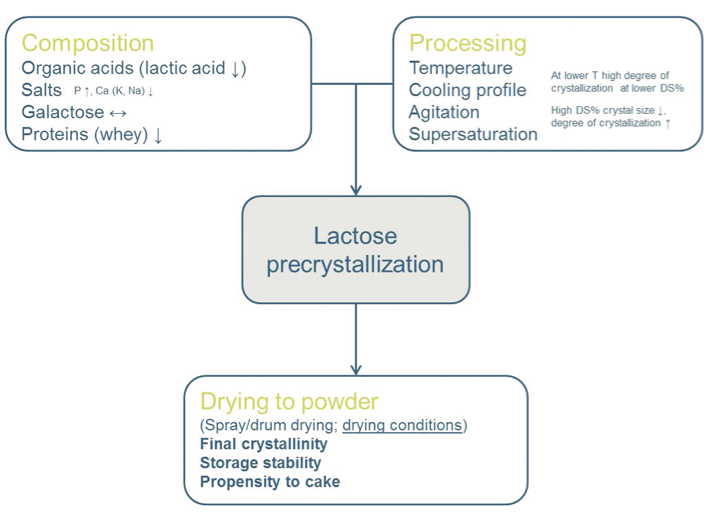
Figure 1: Schematic overview of composition and processing factors affecting pre-crystallisation of lactose in acid whey and the final powder properties (retard (↓) or increase (↑) pre-crystallisation).
Understanding how the different whey constituents affect lactose solubility and crystallisation is thus important for efficient acid whey processing into non-hygroscopic powders.2 For instance, when considering acid whey from yoghurt manufacture (Table 1), high levels of lactic acid, calcium and phosphate are found compared to that of cheese whey. These compositional differences will affect lactose solubility, but also other properties such as viscosity. Concentration will also exceed solubility of calcium lactate, which tends to form needle-shaped crystals and can strongly increase viscosity. Lactic acid is not the only component that affects crystallisation. Minerals also influence the crystallisation rate. There is not only a difference between divalent ions and monovalent ions. For instance, potassium phosphate retards crystallisation, whereas sodium phosphate promotes crystallisation. This can be related to the effects of the different ions on the hydrogen-bonding structure in water and, thereby, the lactose solubility and free energy at the crystal-solvent interface.
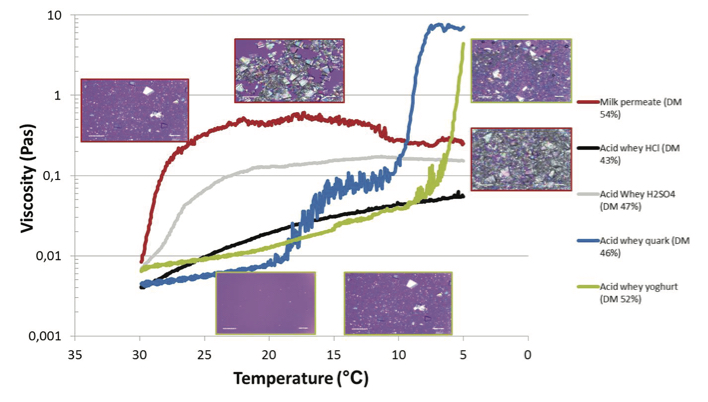
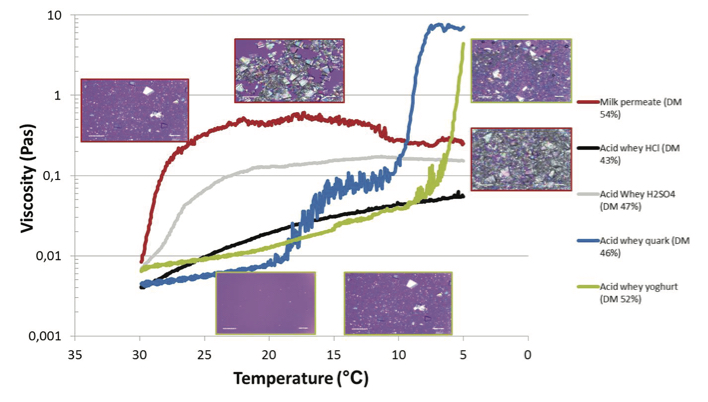
Figure 2: Evolution of viscosity as a function of time (min) during stirring of milk permeate and acid whey concentrates from yoghurt during a cooling cycle from 30 to 5°C in 12h. Micrographs of milk permeate (red) and acid yoghurt whey (green) sampled during crystallisation are shown.
The crystallisation process of various acid whey permeates was evaluated after concentration to ~50% total solids. The induction time of crystallisation and subsequent strong build-up of viscosity of the various acid whey sources was followed in time using microscopy and rheology, respectively, upon applying a cooling cycle. The results of milk permeate and acid whey types are shown in Figure 2.
The induction time of crystallisation and, subsequently, the strong increase in viscosity of acid whey was retarded compared with that of milk permeate. One of the major hindering factors is lactic acid. This is explained by the fact that lactose is surrounded by a strong hydration layer consisting of lactic acid and H3O+ ions via strong H bonds, which restrict water mobility and/or induce a change in structure of lactose, or both, creating unfavourable conditions for lactose crystallisation.3
Acid whey showed an extensive build-up of viscosity compared to that of milk permeate. Moreover, the two types of lactic acid whey (yoghurt and quark whey) showed a further difference in time at which viscosity build up started related to differences in composition. Comparing all acid whey types clearly shows that the acid whey containing HCl or H2SO4 shows behaviour more or less comparable to milk permeate. The extensive build-up of viscosity is related to the occurrence of calcium lactate crystals related to supersaturation of calcium lactate. The generation of calcium lactate crystals shows a large dependence on calcium and lactate contents in combination with pH, and phosphate concentration.4
Other parameters affecting crystallisation are related to the cooling rate and temperature, size and shape of the crystallisation tank and, in particular, agitation. Where these factors might be relatively constant and controlled, the whey composition is highly variable, which makes it difficult to anticipate in process optimisation for further processing.
Lactose crystallisation and powder properties
Lactose crystallisation is not only strongly affected by composition (as shown in Figure 3), but also drying conditions are an important factor. The acid whey concentrate requires sufficient pre-crystallisation to generate a powder with crystalline lactose and minimal amount of amorphous lactose, particularly given that the presence of lactic acid will reduce the glass transition temperature of the amorphous matrix considerably and hence increase the risk of stickiness or caking. This was studied on a large scale to further understand the process-product interactions for acid whey. A trial was carried out in the Processing Centre using quark whey, which was pasteurised, concentrated by evaporation, then flash cooled to ~30°C before crystallisation.
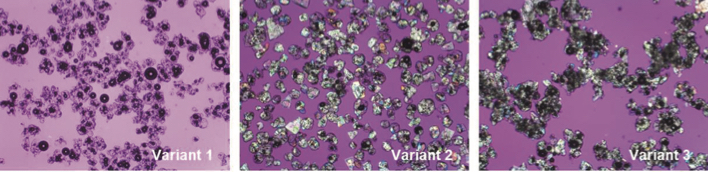
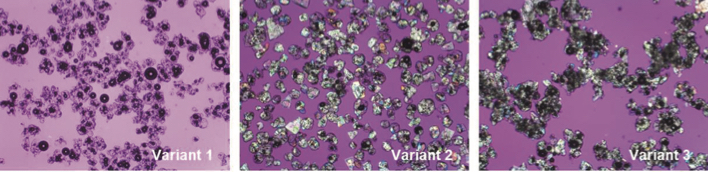
Figure 3: Micrographs of acid quark whey powder as affected by the applied process which affected crystallisation behavior. Picture size 1.9×1.4mm. Samples ranging from a powder without pre-crystallisation of concentrate (variant 1) to a medium (variant 2) and high degree of pre-crystallisation of concentrate (variant 3).
Three ‘model’ powder types were produced ranging from a powder with little or no crystallinity (no pre-crystallisation of concentrate) to a high degree of crystallinity in the powder (sufficient pre-crystallisation of concentrate) (Figure 3). Powder properties were characterised after spray-drying.
The microscopic images of the powders highlight considerable differences in crystallinity of the powders. Whereas variants 2 and 3, where a pre-crystallisation step was applied during processing, clearly show the presence of crystalline material by polarised light microscopy, variant 1, where no pre-crystallisation was applied, showed far less crystalline material. Samples also varied in particle size distribution, as is apparent from microscopic images, but also from complementary light scattering measurements. Particle size and shape, as well as the presence of amorphous lactose, can strongly affect particle interactions, as encountered, for example, during flow of powders.
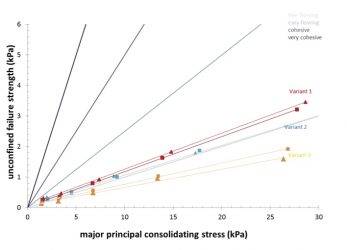
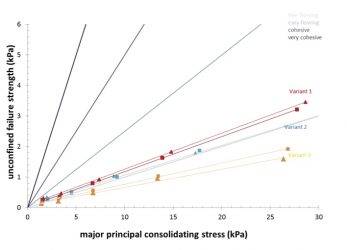
Figure 4: Flow properties of acid quark whey powders as affected by the applied process which affected crystallisation behavior. Samples ranging from a powder without pre-crystallisation of concentrate (variant 1) to a medium (variant 2) and high degree of pre-crystallisation of concentrate (variant 3).
The flowability results shown in Figure 4 highlight that the sample with the highest level of amorphous lactose based on microscopic images had the poorest flow behaviour. This could not be attributed to differences in particle size and shape, but most likely results from the presence of amorphous lactose. When the glass transition temperature of the amorphous matrix is exceeded, the particle can become sticky. While this is typically not expected for pure amorphous lactose under the conditions of measurement, the presence of high levels of lactic acid in the matrix will strongly reduce glass transition temperature and increase chances of a sticky particle surface, and associated impaired flowability.
Overall, the free flowing properties of the three types of acid quark whey powders are affected by differences in applied processing as they all have a similar chemical composition. The different processes changed the free flowing properties which are essentially related to a combination of particle size distribution, particle shape and particle interactions.
Conclusions
Improvement of whey (permeate) powders of industrially available inhomogeneous whey streams requires understanding of the complex interactions of whey composition and applied processing. It is a pre-requisite to know the composition of the whey as a variation in lactose content and type and amount of acid largely influence crystallisation behaviour. This needs to be carefully considered before designing a process and will be helpful in reducing production costs of acid whey powder. Broadening the application area towards food or ingredient applications will assist in the economic feasibility of acid whey powders. Overall, the quality of the acid whey powder (eg, absence of caking, good solubility, no discolouration) will affect the quality of the product in which the acid whey is applied.
References
- Codex standard for whey powders CODEX STAN 289-1995. Formerly CODEX STAN A-15-1995. Adopted in 1995. Revision 2003. Amendment 2006, 2010
- Huppertz, T., and Gazi, I. (2015). Lactose in dairy ingredients: Effect on processing and storage stability. Journal of Dairy Science 98:1-10
- Wijayasinghe, R., Vasiljevic, T., and Chandrapala, J. (2015). Water-lactose behaviour as a function of concentration and presence of lactic acid in lactose model systems. Journal of Dairy Science 98:8505-8514
- Mimouni, A., Bouhallab, S., Famelart, M.H., Naegele, D. and Schuck, P. (2007). The formation of calcium lactate crystals is responsible for concentrated acid whey thickening. Journal of Dairy Science 90:57-65.