Membrane and microchannel emulsification
- Like
- Digg
- Del
- Tumblr
- VKontakte
- Buffer
- Love This
- Odnoklassniki
- Meneame
- Blogger
- Amazon
- Yahoo Mail
- Gmail
- AOL
- Newsvine
- HackerNews
- Evernote
- MySpace
- Mail.ru
- Viadeo
- Line
- Comments
- Yummly
- SMS
- Viber
- Telegram
- Subscribe
- Skype
- Facebook Messenger
- Kakao
- LiveJournal
- Yammer
- Edgar
- Fintel
- Mix
- Instapaper
- Copy Link
Posted: 4 July 2012 | Dr Henelyta Ribeiro, Global Project Leader, Unilever | No comments yet
The production of emulsions with very low mechanical stress and a lower energy input than conventional mechanical methods have been developed in recent years and will be presented in this overview, the so-called membrane and microchannel emulsification. They are efficient processes due to their operational flexibility, reproducibility, straightforward upscaling and reduction in the ratio of equipment size to production capacity, which may lead to novel process intensification1.
The literature has reported distinct membrane and microchannel emulsification technologies developed in various laboratories around the world (Japan, Germany, UK, Switzerland, the Netherlands, France and USA). Membrane emulsification methods include cross-flow, dead-end (also called premix), rotating and vibrating processes. Microchannel emulsifica – tion can be performed using T and Y-junctions, and also by multiple microchannels as the grooved and asymmetric straight-through array types2. Both technologies are able to produce monodisperse oil-in-water (O/W) and water-inoil (W/O) emulsions. It depends on the surface hydrophilicity or hydrophobicity of the membrane respectively. Multiple emulsions (W/O/W) with uniform droplet sizes can also be successfully manufactured.
The production of emulsions with very low mechanical stress and a lower energy input than conventional mechanical methods have been developed in recent years and will be presented in this overview, the so-called membrane and microchannel emulsification. They are efficient processes due to their operational flexibility, reproducibility, straightforward upscaling and reduction in the ratio of equipment size to production capacity, which may lead to novel process intensification1. The literature has reported distinct membrane and microchannel emulsification technologies developed in various laboratories around the world (Japan, Germany, UK, Switzerland, the Netherlands, France and USA). Membrane emulsification methods include cross-flow, dead-end (also called premix), rotating and vibrating processes. Microchannel emulsifica - tion can be performed using T and Y-junctions, and also by multiple microchannels as the grooved and asymmetric straight-through array types2. Both technologies are able to produce monodisperse oil-in-water (O/W) and water-inoil (W/O) emulsions. It depends on the surface hydrophilicity or hydrophobicity of the membrane respectively. Multiple emulsions (W/O/W) with uniform droplet sizes can also be successfully manufactured.
The production of emulsions with very low mechanical stress and a lower energy input than conventional mechanical methods have been developed in recent years and will be presented in this overview, the so-called membrane and microchannel emulsification. They are efficient processes due to their operational flexibility, reproducibility, straightforward upscaling and reduction in the ratio of equipment size to production capacity, which may lead to novel process intensification1.
The literature has reported distinct membrane and microchannel emulsification technologies developed in various laboratories around the world (Japan, Germany, UK, Switzerland, the Netherlands, France and USA). Membrane emulsification methods include cross-flow, dead-end (also called premix), rotating and vibrating processes. Microchannel emulsifica – tion can be performed using T and Y-junctions, and also by multiple microchannels as the grooved and asymmetric straight-through array types2. Both technologies are able to produce monodisperse oil-in-water (O/W) and water-inoil (W/O) emulsions. It depends on the surface hydrophilicity or hydrophobicity of the membrane respectively. Multiple emulsions (W/O/W) with uniform droplet sizes can also be successfully manufactured.
The cross-flow or direct membrane emulsification process is the most conventional one applied on the production of mono – dispersed O/W, W/O emulsions, and also multiple emulsions. This process requires relatively low pressures to force the disperse phase to pass through the membrane pores and detach from the membrane into the continuous phase which contains an emulsifier to stabilise the formed droplet. Pore diameter of the membrane can vary from submicron to micro size ranges, and they are very important to determine the final droplet size of the emulsion. Uses of membrane with a pore diameter smaller than 0.4 μm can limit production rates due to strong fouling.
Premix membrane emulsification or the dead-end technique was developed in Japan in 19982. In this process, a pre-emulsion with large droplet sizes is passed through the pores of the membrane by low pressure. The mother droplets from the pre-emulsion are disrupted into fine daughter droplets during their permeation through the membrane. The generated droplet sizes are larger than the mean pore size of the membrane. Passing the resulting emulsion through the membrane several times can result in a smaller mean droplet size and narrower droplet size distribution. In the deadend technique, the dispersed phase fraction is independent of the process time, which is an advantage over cross-flow membrane emulsification. Although the premix membrane emulsification process is easier to operate and control than cross-flow membrane emulsification, fouling of the membrane can be a severe problem.
In the rotating membrane emulsification, droplets are generated after radially forcing the disperse phase through the pores of a rotating cylindrical membrane into the continuous phase containing an emulsifier. This technique has potential for industrial application as it substantially increases the flow rate of the dispersed phase through the pores of the membrane. Vibrating membrane emulsification is still under development. It is better suited for applications where flat membranes, such as perforated metal plates and micro-engineered silicon wafers are applied.
In the 1990s, Professor Nakajima and his research group developed microchannel emulsification to allow the production of highly uniform droplets with a small coefficient of variation of below five per cent3. For the droplet generation, grooved and straight-through microchannel arrays were designed. Using this technique, the droplets are generated under very mild conditions and high energy efficiency. Grooved microchannel plates allow the production of smaller droplets than the straightthrough type, however the last one consisting of highly integrated microfluidic through-holes remarkably improves the droplet productivity and decreases the coefficient of variation to numbers below five per cent. Microchannel plates are made of silicon and need special surface treatment for the production of water or oil continuous dispersions. Microchannel emulsification currently produces droplets only above one micrometre size.
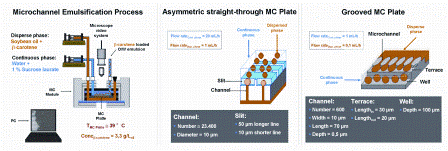
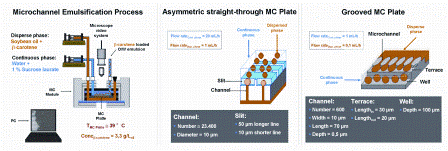
Figure 1: Schematic drawings of the microchannel emulsification (MC) process and the droplet generation through a straight-through MC array and grooved MC array
Figure 1 illustrates a schematic microchannel emulsification process and the two types of microchannel plates currently used on the production of microstructures. The generation of β-carotene-loaded oil droplets was captured using a high-speed camera and an optical microscope (Figure 2).
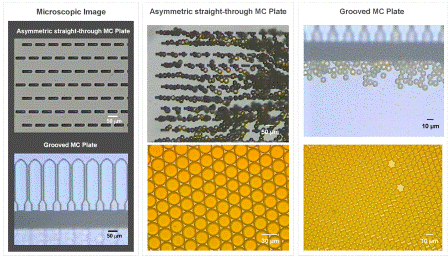
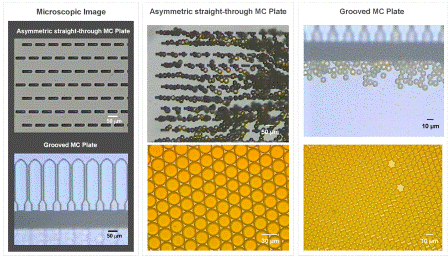
Figure 2: Microscopic images of straight-through MC and grooved MC array, and the droplet formation. Example of oil-in-water emulsion production
Applications
The food industry aims to manufacture products with high nutritional value, natural taste and excellent overall quality. Low shear processes are more appropriate as they can preserve intact sensitive ingredients (e.g. flavours, enzymes, microorganisms, antioxidants, etc.), and consequently allow the development of more tasty and healthier food products.
From an application point of view, membrane and microchannel emulsification processes have attracted the attention of various industries due to their potential to produce very narrow droplet size distributions compared to other emulsification techniques. Single and multiple emulsions, solid lipid microparticles, gel particles (calcium alginate), core shell particles, protein microspheres and complex coacervate microcapsules can be produced under relatively mild conditions. A very interesting and appropriate application can be addressed to parenteral emulsions as droplet size distribution can be easily controlled by process parameter. Also, the production of sub- and microbubbles via cross-flow membrane emulsification has shown some advantages2. Figure 3 shows some applications of microchannel emulsification for the food and pharmaceutical industry. Monodisperse emulsions produced by microchannel emul – sification have been also used as templates for obtaining monodisperse microdispersions such as microparticles and microcapsules. Gel microbeads and giant vesicles were fabricated via uniform water droplets and have been used as carrier systems for functional active molecules for food applications. Monodisperse giant vesicles could also be applied in the pharmaceutical industry as phospholipids are used in their stabilisation.
Multiple emulsions can be easily produced using membrane and microchannel emul – sification. They are very interesting for application as a delivery system of (bio)active compounds and for the production of low fat food products such as salad dressings, mayonnaise and sausages. The drawback is their short physical stability, which limits their application in the industry.
For all types of applications, wettability of the membrane plays an important role on the positive performance of droplets production and their size distribution3. Before starting the emulsification process, the membrane always needs to be wetted by the continuous phase.
Key considerations
This short review has shown the pros and cons of using membrane and microchannel emulsification for food applications. They are certainly innovative processes which can be easily implemented at factory scale at lower capital costs compared to conventional emulsification processes. However, it is important to highlight that running costs can be higher than high pressure homogenisation processes and rotor-stator systems due to long cleaning procedures that need to be applied.
Advantages on the production of narrow droplet size distribution can be addressed more for pharmaceutical application than for the food industry where physical stability of emulsion-based food products can be easily managed with the application of hydrocolloids. However, a potential application of membrane and microchannel emulsification in the food industry is on the production of multiple emulsions if they can be kept physically stable for several months. It would lead to a breakthrough on the formulation of new food and nonfood products.
Food products normally have protein in their formulations and they can be adsorbed on the membrane surface leading to changes on its surface (wettability) over time, consequently fouling may take place reducing the production time. Perhaps the most important consideration for these technologies is still the necessity of a deep understanding of surface property of the different type of membranes, also the development of new surface properties since fouling is a big issue to move these processes forward to industrial application, where very high flow rates is required.
References
1. Charpentier J.-C. 2007. Modern chemical engineering in the framework of globalization, sustainability, and technical innovation. Ind. Eng. Chem. Res, 46, 3465-3485
2. Ribeiro HS, Janssen JJM, Kobayashi I, Nakajima M. 2010. Membrane Emulsification for Food Applications, in Membranes for Food Treatment, K-V Peineman, S Nunes, L Giorno (Eds.), Wiley-VCH Ed., Weinheim
3. Kobayashi I, Nakajima M. 2006. Generation and multiphase flow of emulsions in microchannels. in Micro Process Engineering, N. Kockmann (Ed.), Wiley-VCH, Weinheim
4. Sugiura S, Nakajima M, Tong J, Nabetani H, Seki M. 2000. Preparation of monodispersed solid lipid microspheres using a microchannel emulsification technique. J. Colloid Interface Sci., 227, 95-103
5. Kawakatsu T, Oda N, Yonemoto T, Nakajima M. 1999. Production of monodispersed albumin gel microcapsules using microchannel W/O emulsification. Kagaku Kogaku Ronbunshu, 26, 122-125
6. Kobayashi I, Lou X, Mukataka S, Nakajima M. 2005. Preparation of monodisperse water-in-oil-in-water emulsions using microfluidization and straightthrough microchannel emulsification. J. Am. Oil Chem. Soc., 82, 65-71
7. Kuroiwa T, Kikuchi H, Noda K, Kobayashi I, Nakajima M, Uemura K, Sato S, Mukataka S, Ichikawa S. 2009. Controlled preparation of giant vesicles from uniform water droplets obtained by microchannel emulsification with bilayer-forming lipids as emulsifiers. Microfluid. Nanofluid., 6, 811-821
8. Nakagawa K, Iwamoto S, Nakajima M, Shono A, Satoh K. 2004. Microchannel emulsification using gelatin and surfactant-free coacervate microencapsulation. J. Colloid Interface Sci., 278, 198-205
9. Xu QY, Nakajima M, Binks BP. 2005. Preparation of particle-stabilized oil-in-water emulsions with the microchannel emulsification method. Colloids and Surface A: Physicochemical and engineering aspects, 262, 94-100
10. Chuah AM, Kuroiwa T, Kobayashi I, Nakajima M. 2009. Effect of chitosan on the stability and properties of modified lecithin stabilized oil-in-water monodisperse emulsion prepared by microchannel emulsification. Food Hydrocolloids, 23, 600-610
About the author
Dr. Henelyta Ribeiro is a Chemical Engineer by training, having obtained a MSc and PhD in Food Process Engineering. From 2001 to 2004, she worked as a Research Scientist in Emulsification at the Institute of Food Process Engineering in the University of Karlsruhe, Germany. In 2005-2006, Henelyta did a Post-Doc at the National Food Research Institute in Japan in collaboration with Tsukuba University, on the production of O/W emulsions containing lipophilic bioactive compounds by microchannel emulsification and emulsification diffusion methods. Henelyta joined Unilever R&D Colworth in the UK as a Research Scientist in Particulate Structuring in October 2006. After working on a number of areas including Processing and Novel Microstructures, Henelyta moved to Unilever R&D Vlaardingen in the Netherlands and is currently working as a Global Project Leader.