New thoughts on a time-tested technology: milk pasteurisation
- Like
- Digg
- Del
- Tumblr
- VKontakte
- Buffer
- Love This
- Odnoklassniki
- Meneame
- Blogger
- Amazon
- Yahoo Mail
- Gmail
- AOL
- Newsvine
- HackerNews
- Evernote
- MySpace
- Mail.ru
- Viadeo
- Line
- Comments
- Yummly
- SMS
- Viber
- Telegram
- Subscribe
- Skype
- Facebook Messenger
- Kakao
- LiveJournal
- Yammer
- Edgar
- Fintel
- Mix
- Instapaper
- Copy Link
Posted: 5 September 2012 | Daina Ringus and Kathryn Boor, College of Agriculture and Life Sciences, Food Science Department, Cornell University | No comments yet
The commercial adoption of milk pasteurisation was a major boon for urban public health in the first half of the 20th century. Before the widespread use of pasteurisation, the proliferation of diseases such as bovine tuberculosis and brucellosis among humans was frequently linked with consumption of unpasteurised (raw) milk15. Pasteurisation remains an important public health measure since raw milk can transmit pathogens associated with animals. For example, in the past year in the United States, human foodborne outbreaks caused by Salmonella and Campylobacter jejuni have been linked to consumption of raw milk.
Pasteurisation is a heat treatment applied to inactivate all non-spore-forming human pathogens associated with milk. Pasteurisation is not intended to sterilise milk, but rather to inactivate harmful bacteria. Current processing time and temperature requirements are designed to destroy Coxiella burnetii, the causative agent of Q fever. This organism is considered to be the most heat-resistant human pathogen associated with milk6. Continuous high temperature, short time (HTST) pasteurisation is the most commonly used method for pasteurising fluid milk in the US. HTST pasteurisation requires heating to 72°C for 15 seconds, as recommended by the Grade ‘A’ Pasteurised Milk Ordinance (PMO), the basis for dairy regulatory programs in the US1. The same HTST time/temperature requirements are applied in Australia3, with a similar combination, 71.7°C for 15 seconds, in Europe4. Milk can become contaminated with microorganisms before or after pasteurisation.
The commercial adoption of milk pasteurisation was a major boon for urban public health in the first half of the 20th century. Before the widespread use of pasteurisation, the proliferation of diseases such as bovine tuberculosis and brucellosis among humans was frequently linked with consumption of unpasteurised (raw) milk15. Pasteurisation remains an important public health measure since raw milk can transmit pathogens associated with animals. For example, in the past year in the United States, human foodborne outbreaks caused by Salmonella and Campylobacter jejuni have been linked to consumption of raw milk. Pasteurisation is a heat treatment applied to inactivate all non-spore-forming human pathogens associated with milk. Pasteurisation is not intended to sterilise milk, but rather to inactivate harmful bacteria. Current processing time and temperature requirements are designed to destroy Coxiella burnetii, the causative agent of Q fever. This organism is considered to be the most heat-resistant human pathogen associated with milk6. Continuous high temperature, short time (HTST) pasteurisation is the most commonly used method for pasteurising fluid milk in the US. HTST pasteurisation requires heating to 72°C for 15 seconds, as recommended by the Grade ‘A’ Pasteurised Milk Ordinance (PMO), the basis for dairy regulatory programs in the US1. The same HTST time/temperature requirements are applied in Australia3, with a similar combination, 71.7°C for 15 seconds, in Europe4. Milk can become contaminated with microorganisms before or after pasteurisation.
The commercial adoption of milk pasteurisation was a major boon for urban public health in the first half of the 20th century. Before the widespread use of pasteurisation, the proliferation of diseases such as bovine tuberculosis and brucellosis among humans was frequently linked with consumption of unpasteurised (raw) milk15. Pasteurisation remains an important public health measure since raw milk can transmit pathogens associated with animals. For example, in the past year in the United States, human foodborne outbreaks caused by Salmonella and Campylobacter jejuni have been linked to consumption of raw milk.
Pasteurisation is a heat treatment applied to inactivate all non-spore-forming human pathogens associated with milk. Pasteurisation is not intended to sterilise milk, but rather to inactivate harmful bacteria. Current processing time and temperature requirements are designed to destroy Coxiella burnetii, the causative agent of Q fever. This organism is considered to be the most heat-resistant human pathogen associated with milk6. Continuous high temperature, short time (HTST) pasteurisation is the most commonly used method for pasteurising fluid milk in the US. HTST pasteurisation requires heating to 72°C for 15 seconds, as recommended by the Grade ‘A’ Pasteurised Milk Ordinance (PMO), the basis for dairy regulatory programs in the US1. The same HTST time/temperature requirements are applied in Australia3, with a similar combination, 71.7°C for 15 seconds, in Europe4. Milk can become contaminated with microorganisms before or after pasteurisation. Prior to processing, commensal bacteria from cows’ bodies, bacteria from infected cows, and bacteria from the farm can enter raw milk. Postpasteurisation contamination of pasteurised milk can occur during processing, packaging, storage and distribution of product. For example, milk filler heads can harbour bacteria that can contaminate freshly pasteurised milk.
The PMO stipulates that grade ‘A’ pasteurised milk has a Standard Plate Count (SPC) < 20,000 cfu/ml and ≤10 cfu/ml of coliforms throughout its shelf life1. Consumers notice that milk is ‘spoiled’ when microbes grow to high enough numbers to cause an undesirable breakdown of its components. The resulting organoleptic changes decrease consumer acceptability of milk products. Therefore, in order to extend shelf life, dairy processors must work to reduce bacterial contamination of pasteurised milk.
The Cornell University Milk Quality Improvement Program (MQIP) seeks to improve the quality and safety of dairy products in New York State. Some of the recent research conducted by our group includes studying (i) the microbiological ecology of raw and pasteurised milk, (ii) the effects of HTST pasteurisation on milk quality during shelf life, and (iii) evaluating microbiological tests used by the dairy industry to assess milk quality. The following findings represent the MQIP’s recent contributions to understanding the impact of pasteurisation on the microbiology of milk.
The ecology of milk
Two general classes of spoilage organisms are found in milk: psychrotrophic Gram-negative bacteria and Gram-positive organisms. Psychrotrophic Gram-negative bacteria, such as Pseudomonas spp., are not heat-resistant. Therefore, their presence in pasteurised milk reflects their entry post-pasteurisation, via contaminated processing equipment7. Psychro – trophic organisms can grow at refrigeration temperatures (0-7°C); hence, they may reach high numbers in milk during storage. Many Pseudomonas spp. secrete extracellular enzymes such as proteases, lipases and lecithinases. These enzymes degrade protein, fats and fat globule membranes, respectively, which can result in bitter, rancid and coagulated milk5. Imple – menting strategies to reduce bacterial postpasteurisation contamination has allowed processors to extend shelf life2.
Gram-positive bacteria that can spoil pasteurised milk can enter milk pre- or postprocessing. Some of these organisms, such as Bacillus spp. and Paenibacillus spp., can form heat-resistant spores that survive pasteurisation. Spores are a dormant, non-reproducing cell type of some Firmicutes that are resistant to extreme conditions, including UV irradiation, nutrient starvation, and high and low temperatures.
The presence of Gram-positive spore forming members of the genera Bacillus and Paenibacillus is the current biological barrier in extending the shelf life of HTST fluid milk. Studies tracking milk throughout the supply chain, from raw milk tank trucks to packaged product, have identified multiple possible entry points for these bacteria. DNA sequencing of genes conserved among all bacteria, i.e., rpoB and 16S DNA genes, allows discrimination among bacterial subtypes. Data from such analyses has been used to track transmission of specific bacterial strains throughout the supply chain. For example, the same subtypes of Paenibacillus spp. and Bacillus spp. have been detected in both raw milk and in pasteurised, refrigerated milk8, showing that entry into raw milk by these organisms is an important contamination point that can lead to reduced shelf life after pasteurisation.
Pasteurisation survivors: Paenibacillus and Bacillus spp.
In the absence of post-pasteurisation contamination by Gram-negative bacteria, an ecological shift occurs in Gram-positive spoilage organisms in milk during refrigerated shelf life. While Bacillus spp. are generally the most commonly isolated Gram-positive bacteria obtained from refrigerated pasteurised milk for the first 14 days of shelf life, Paenibacillus spp. become the dominant species after this period. This ecological shift reflects the fact that, in general, Paenibacillus spp. are more psychro – tolerant than Bacillus spp. For example in one study, Gram-positive spore forming isolates were collected and characterised from laboratory-pasteurised milk over 21 days of refrigeration. On day one, 91 per cent of the isolates characterised were Bacillus spp., compared to only nine per cent Paenibacillus. After 21 days of refrigeration, however, only five per cent of the isolates were Bacillus spp., while 95 per cent were Paenibacillus spp.14 We have observed similar shifts in dominant bacteria in pasteurised milk samples collected from dairy plants throughout the US13.
Our group has begun to characterise psychrotolerant spore forming spoilage microbes. We have found that a majority of dairy-associated Paenibacillus grow in skim milk broth at 6°C, compared to a majority of Bacillus that do not. Furthermore, a majority of these dairy-associated Paenibacillus spp. demonstrate β-galactosidase activity – the ability to enzymatically breakdown lactose sugars – at 32°C compared to most Bacillus isolates that do not9. These findings suggest mechanisms that may explain the dominance of Paenibacillus in pasteurised milk at the end of shelf life.
Our work characterising the ecology and diversity of bacteria found in fluid milk indicates that when post-pasteurisation contamination has been controlled or eliminated, Paenibacillus spp. become the primary spoilage organisms that limit fluid milk shelf life. A better understanding of the Paenibacillus spp. ecology and genetic diversity will aid our efforts to extend the shelf life of pasteurised milk.
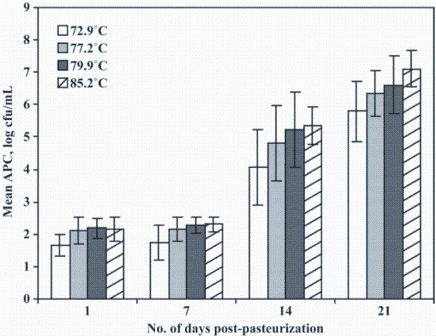
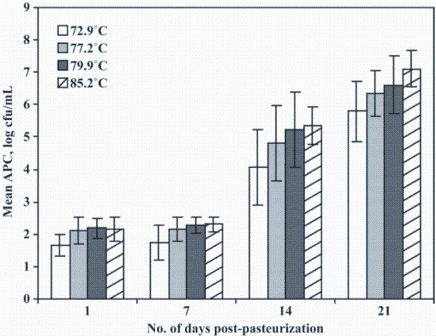
Figure 1: FIGURE 1 Bacterial counts of HTST-processed fluid milk at different temperatures. Aerobic plate counts (APC) for 2 per cent fat milk that had been pasteurised at one of four different temperatures (72.9, 77.2, 79.9, or 85.2ºC) and held at 6ºC for up to 21 days. Data represent mean APC for milk processed at each temperature from four independent replicates. Bars indicate mean ± standard deviation for each treatment
For quality, temperature matters: effects of HTST temperature on the shelf life of pasteurised fluid milk
Many dairy processors in the US pasteurise milk at temperatures above the HTST minimum temperature. Anecdotal evidence suggests processors believe higher temperatures will lead to increased food safety and longer shelf life. While perhaps at first counterintuitive, recent research has shown that higher HTST pasteurisation temperatures lead to higher bacterial counts and reduced shelf life of HTST milk during refrigerated storage.
In an experimental study, we tested the effect of four different HTST temperatures on the microbiological quality of pasteurised milk over shelf life. In general, we observed higher bacterial outgrowth in milk pasteurised at higher temperatures. Milk (two per cent fat) was pasteurised at 72.9, 77.2, 79.9, or 85.2°C, for 25 seconds. The milk was refrigerated, and sampled after one, seven, 14 and 21 days of refrigerated storage for total aerobic bacterial numbers (aerobic plate counts). On all four days, the mean bacterial numbers were significantly lower in milk heated to 72.9°C rather than to 85.2°C (Figure 1). We characterised isolates from the pasteurised milk samples to determine if these HTST-processing temperatures differentially affected Paenibacillus spp. or Bacillus spp. Statistical analysis of the microbiological data showed that the various HTST temperatures did not affect members of these genera differently.
To test whether higher HTST temperatures would reduce the shelf life of milk processed in a commercial operation, we conducted a follow up case study with one New York State milk processor11. The processor voluntarily lowered its HTST pasteurisation temperature to 76.1°C for 15 months after processing at 79.4°C for 15 months. Microbiological analyses of pasteurised milk samples held at 6°C over 21 days of shelf life confirmed the previous study’s findings. In the case study, the mean bacterial counts, as determined by SPC, of the milk heated to the higher temperature were significantly higher on all days compared to the milk pasteurised at the lower temperature.
These studies show that higher pasteurisation temperatures can lead to reduced shelf life of HTST-processed fluid milk. We have proposed two hypotheses that may explain this effect. First, higher HTST temperatures approach spores’ optimal germination temperature of 80°C12, which may lead to a higher number of germinated spores that continue to grow in HTST processed fluid milk pasteurised at higher temperatures. Another possibility may be that the greatest inactivation of lactoperoxidase, a bacteriostatic enzyme naturally present in milk that is heat-labile, occurs at <80°C. Further work understanding this mechanism will greatly contribute to our ability to limit the growth of spoilage organisms in HTST pasteurised milk.
No crystal ball for shelf life: raw milk tests on high quality grade A pasteurised milk do not predict fluid product shelf life
The dairy industry uses several microbiological tests to assess raw milk quality. These tests include the standard plate count (SPC), psychrotrophic bacteria count (PBC), coliform count (CC), laboratory pasteurisation count (LPC), direct microscopic count, and the preliminary incubation (PI) count. The PI test in particular has become increasingly used by processors to differentiate the quality and potential negative impact of raw milk on their products. In this test, raw milk is held at 55°C for 18 hours before plating onto SPC agar plates then incubated at 32°C for 48 hours. The prevailing logic for this test presumes that bacteria from healthy cows will not reproduce at 55°C, while other contaminating bacteria – introduced by poor hygiene during raw milk handling – will replicate and be detected by the test.
To determine if raw milk microbiological tests accurately predict fluid milk shelf life, we evaluated raw and HTST-processed fluid milk from four New York State milk processing plants using these tests10. The milk used in this study was high quality grade ‘A’ milk, as determined by bacterial load. For example, 76 per cent of the raw milk samples had SPC counts <10,000 cfu/ml, an indicator of high-quality grade ‘A’ milk. We sought to determine the validity of using raw milk microbiological tests to predict shelf life after pasteurisation of high quality raw milk.
We performed the six microbiological tests mentioned above, and four others, including the ropy milk test and plating onto media that select for or differentiate Streptococcus spp., Staphylococcus spp., or Gram-negative organisms. Sensory evaluation by a trained panel and microbiological analyses of the pasteurised milk samples were performed over 17 days of refrigerated storage.
Statistical analyses of the results from the individual raw milk microbiological tests found little correlation with the microbiological quality and sensory acceptability of the pasteurised milk over shelf life. Several of these tests, such as plating onto crystal violet tetrazolium agar and the PBC test, select for psychrotrophic Gramnegative organisms such as Pseudomonas spp., which are common post-pasteurisation con – taminants. In our study, the results of the raw milk quality tests failed to show a relationship between test results from the microbiological tests and the quality of milk pasteurised from the same raw milk. These results indicate that raw milk tests performed on high quality grade ‘A’ raw milk do not accurately predict pasteurised milk quality.
Conclusions
Our work in the MQIP has elucidated the bacterial ecology of raw and HTST-processed fluid milk and has allowed processors to consider bacterial contamination entry points in the milk processing continuum. Our work will focus on development of effective inter – ventions for control or elimination of spoilage organisms from pasteurised milk to increase quality, extend shelf life and reduce waste of a nutritious commodity.
References
1. Food and Drug Administration. 2011 Revision. Grade “A” Pasteurized Milk Ordinance (PMO), U.S. Dept. of Health and Human Services, Public Health Service
2. Carey, N. R., S. C. Murphy, R. N. Zadoks, and K. J. Boor. 2005. Shelf lives of pasteurized fluid milk products in New York State: A ten-year study. Food Protection Trends:102-113
3. Australian New Zealand Food Authority, Food Standards Code. 2006. Primary Production and Processing Standard for Dairy Products, Standard 4.2.4, Division 4.15
4. European Economic Community. Council Directive 92/46/EEC of 16 June 1992 laying down the health rules for the production and placing on the market of raw milk, heat-treated milk and milk-based products. http://eceuropaeu/food/fs/sfp/mr/mr03_enpdf
5. Dogan, B., and K. J. Boor. 2003. Genetic diversity and spoilage potentials among Pseudomonas spp. isolated from fluid milk products and dairy processing plants. Applied and Environmental Microbiology 69:130-138
6. Enright, J. B., W. W. Sadler, and R. C. Thomas. 1957. Thermal inactivation of Coxiella burnetii and its relation to pasteurization of milk. Public Health Monograph 47:1
7. Fromm, H. I., and K. J. Boor. 2004. Characterization of pasteurized fluid milk shelf-life attributes. Journal of Food Science 69:M207-M214
8. Huck, J. R., B. H. Hammond, S. C. Murphy, N. H. Woodcock, and K. J. Boor. 2007. Tracking sporeforming bacterial contaminants in fluid milkprocessing systems. Journal of Dairy Science 90:4872-4883
9. Ivy, R. A., M. L. Ranieri, N. H. Martin, H. C. den Bakker, B. M. Xavier, M. Wiedmann, and K. J. Boor. 2012. Identification and characterization of psychrotolerant sporeformers associated with fluid milk production and processing. Applied and Environmental Microbiology10.1128/AEM.06536-11
10. Martin, N. H., M. L. Ranieri, S. C. Murphy, R. D. Ralyea, M. Wiedmann, and K. J. Boor. 2010. Results from raw milk microbiological tests do not predict the shelf-life performance of commercially pasteurized fluid milk. Journal of Dairy Science 94:1211-1222
11. Martin, N. H., M. L. Ranieri, M. Wiedmann, and K. J. Boor. 2011. Reduction of pasteurization temperature leads to lower bacterial outgrowth in pasteurized fluid milk during refrigerated storage: A case study. Journal of Dairy Science 95:471-475
12. Morn, L., M. T. Row, and J. A. Hagan. 1990. The effect of various heat activation treatments on fast, intermediate and slow germinating spores of Bacillus spp. Letters in Applied Microbiology 10:43-46
13. Ranieri, M. L., and K. J. Boor. 2009. Short communication: Bacterial ecology of hightemperature, short-time pasteurized milk processed in the United States. Journal of Dairy Science 92:4833-4840
14. Ranieri, M. L., J. R. Huck, M. Sonnen, D. M. Barbano, and K. J. Boor. 2009. High temperature, short time pasteurisation temperatures inversely affect bacterial numbers during refrigerated storage of pasteurised fluid milk. Journal of Dairy Science 92:4823-4832
15. Steele, J. H. 1954. Milk sanitation, communicable disease, and public health. Public Health Reports 69:1065
Figure 1 copyright: Copyright: Reprinted from Journal of Dairy Science, Vol. 92, M. L. Ranieri, J. R. Huck, M. Sonnen, D. M. Barbano, and K. J. Boor, High temperature, short time pasteurization temperatures inversely affect bacterial numbers during refrigerated storage of pasteurized fluid milk, 4823-2832, ©2009, with permission from American Dairy Science Association.
About the authors
Kathryn J. Boor is the Ronald P. Lynch Dean of the New York State College of Agriculture and Life Sciences and a Professor in the Department of Food Science at Cornell University. Having a research/extension appointment, she is the director of the Milk Quality Improvement Program and the Food Safety Laboratory. Her research interests include understanding the presence and persistence of spoilage and pathogenic bacteria, such as Listeria monocytogenes, in our food supply.
Daina Ringus is a PhD candidate in Food Science & Technology at Cornell University. She studies the stress response of Listeria monocytogenes. Her dissertation project characterises small molecule inhibitors of the pathogen’s stress response. She has participated in many cheese and dairy education activities throughout her doctoral program.